The James Webb Space Telescope is an outstanding project; it is the biggest, most intricate, and most costly (10 billion USD) device that humanity has ever sent into space. This telescope is able to see more clearly and farther than any other space telescope thanks to its 269 square foot (25 square meter) mirror and extremely sensitive infrared optics. The launch of the James Webb Space Telescope on December 25, 2022, was one of the most challenging tasks ever carried out in space. Because the tennis court-sized sunshield and mirrors had to unfold from their compact transport configuration, which resembled a reverse origami. Also, more than 300 steps and mechanisms had to function with perfect precision.
The James Webb telescope traveled 930,000 mi (1.5 million km) away from Earth to get to its “workplace” after the launch. The new “eye in space” examines the atmospheres of neighboring exoplanets and hunts for indications of life while also looking back to the time of the first stars to provide novel insights into the cosmos.
Why is the James Webb Telescope special?
The Hubble Space Telescope has set the standard for expansive space vistas into far-off space and breathtaking photographs of cosmic events. Its photos have offered vital information for astronomical and astrophysical studies for more than 30 years. But as time goes on, the Hubble is becoming older, and failures are mounting. At least part of Hubble’s responsibilities has been taken over by the James Webb Space Telescope (JWST) which is bigger, sharper, and farther.
Greater, Sharper, and Farther
In many respects, JWST is bigger and more advanced than its more seasoned partner. In comparison to the Hubble, which is about the size of a truck, the JWST is a colossal 236 ft (22 m) long and 129 ft (12 meters) wide. What makes this telescope so precious, though, is its primary mirror, which is even more significant. Its 70 ft (6.50 m) diameter is about three times that of Hubble’s 26 ft (2.40 m) mirror. Such a big mirror was never sent into space. The Webb telescope weighs 6.5 metric tons or 14,300 lbs.
The Webb telescope has a lot more light output and resolution as a consequence. As shown by its “Legacy Field,” the Hubble telescope can still see bright objects 13.3 billion light-years away. On the other hand, the JWST is intended to explore older, further parts of space. Galaxies that formed up to 300 million years after the Big Bang are visible now.
It’s a Specialist
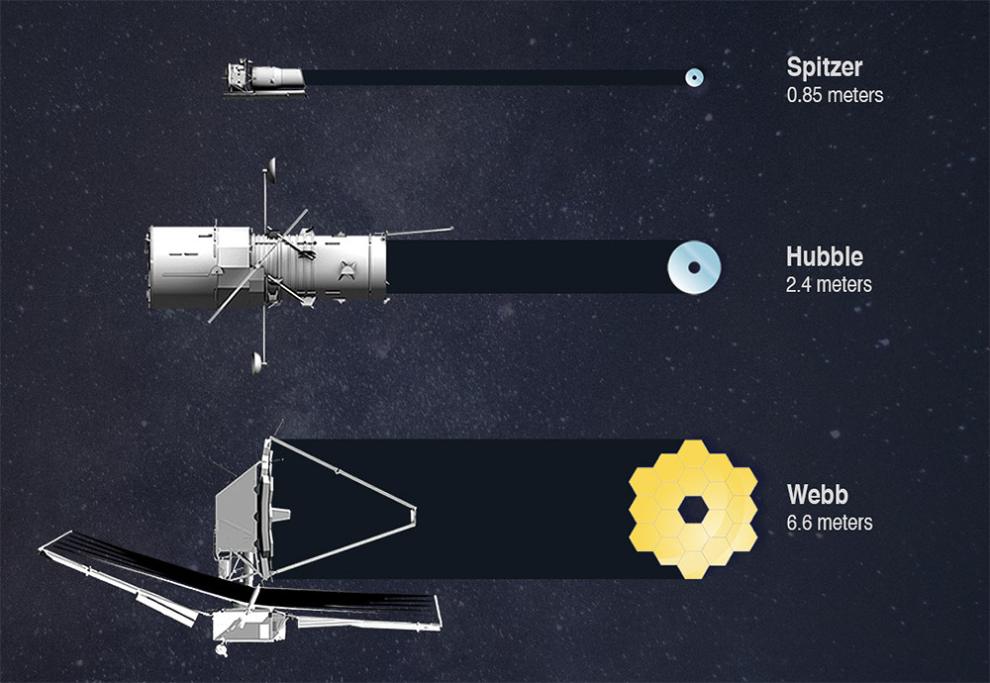
However, since it “sees” a different region of the radiation spectrum than Hubble, the Webb telescope has a distinct perspective on the cosmos. In a way, the Hubble telescope is an all-arounder since its wave spectrum spans from ultraviolet to near-infrared. On the other hand, Webb is more of an expert in infrared: its spectrum spans the mid-infrared range, or 28.5 micrometers to 0.6 micrometers (1122 to 23.6 micro inch), the red zone of visible light that is just visible to humans.
This elevates Webb to the status of a Herschel or Spitzer-style infrared space telescope replacement. The universe has at least as many breathtaking things to offer in infrared as it does in visible light, as their photographs previously showed. The infrared cosmos was made accessible by the breakthrough of the Hubble and Spitzer space telescopes. These missions have now evolved into Webb, which combines Hubble’s sensitivity and resolution with Spitzer’s infrared vision.
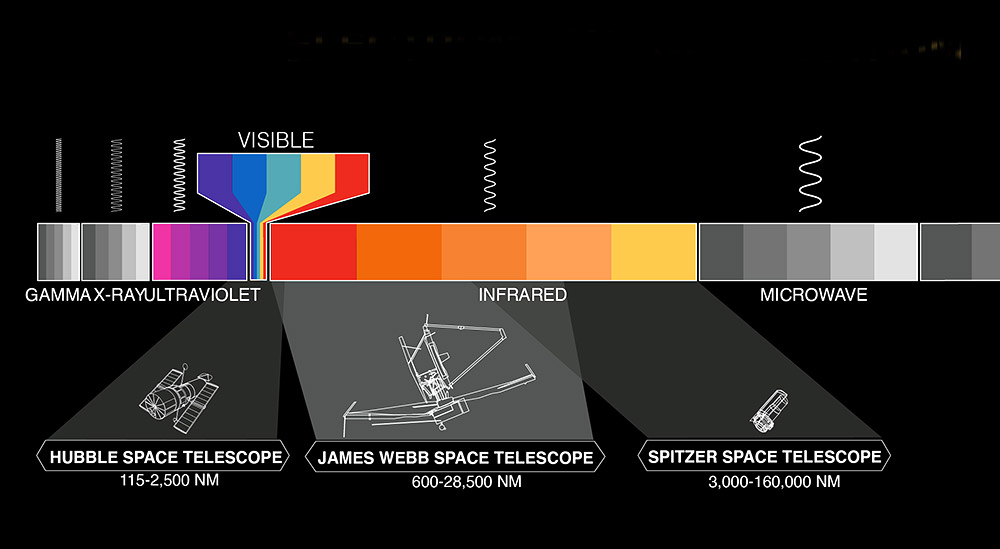
At the same wavelength, Webb’s 0.03 arc second resolution is a little bit sharper than Hubble’s 0.05 arc second. Because of how sensitive its infrared optics are, it is still possible to perceive a single bumblebee’s warmth on the Moon from Earth.
Utilizing the Icy Space
However, the Webb telescope’s very sensitive infrared optics pose additional difficulties. The mirrors and other optics of the JWST must be cooled to practically absolute zero to collect the feeble thermal radiation of the furthest celestial objects. This is the only method to stop the background noise from drowning out the delicate signals in the telescope’s thermal radiation.
As a result, the mirrors and optics of the telescope cannot become any warmer than minus 218 degrees Celsius (-360 Fahrenheit). While Spitzer and other infrared space telescopes have done this by actively cooling their optical components using helium, this is not feasible with the Webb telescope’s massive mirror. As a result, the JWST employs a different tactic: it allows space’s icy air to handle the cooling.
Why the Lagrange Point Instead of Earth’s Orbit?
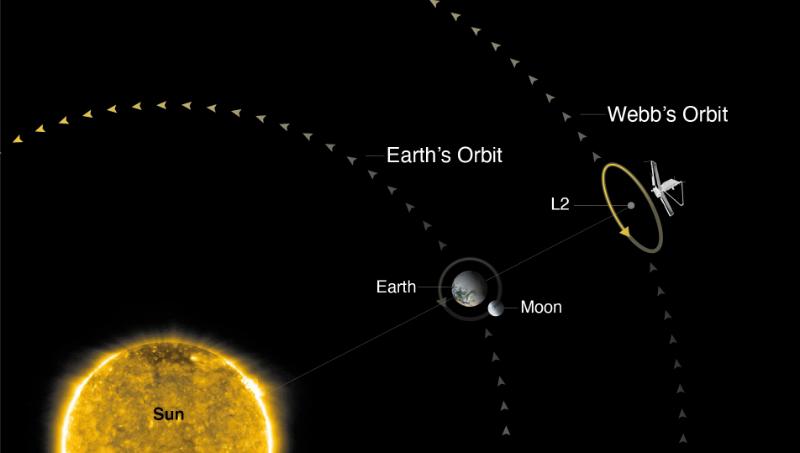
But this also implies that this space telescope cannot orbit the Earth as Hubble can. This is because the Earth emits heat and infrared radiation when seen from space, which would obstruct the observations. In addition, depending on where it is in orbit, the Sun also emits interference radiation. The JWST would need to either have an insulating cover all around, which is impractical due to the large mirror, or a moveable sunshield that would need to be adjusted continuously to entirely shield the optics from every ray of sunlight.
As a result, Lagrange Point 2 has been chosen as the James Webb telescope’s viewing location. This spot is facing away from the Sun and it is 1.5 million km (930,000 mi) from Earth. Space probes may orbit there in a stable manner without using a lot of energy thanks to the equilibrium of the gravitational forces between the Sun and the Earth. One shield is adequate to block the thermal radiation since the sunlight there always arrives from the same direction at the same time. JWST’s thermal shield is the size of a tennis court and is made up of five very thin insulating membranes for the job.
That is why the Webb telescope is neither the first nor the only one at Lagrange Point 2: the no longer operational Wilkinson Microwave Anisotropy Probe (WMAP) and Planck, which have studied the cosmic background radiation, orbit there. The European space telescope Gaia and the X-ray telescope eROSITA are both still operational at the Second Lagrange point (L2).
JWST’s Scientific Objectives
The primary use of infrared light by the James Webb Telescope is directly tied to the scientific objectives of its mission. This is because one of the JWST’s duties is to gaze back in time much farther than Hubble has been able to, to the moment when the very first galaxies were born. Scientists are conducting the first-ever study of galaxies from the immediate post-Big Bang period using Webb. This was not achievable with any other telescope up to JWST.
Since the Big Bang, the universe has been expanding, which causes the radiation that has been traveling to us for billions of years to similarly expand. As a consequence, the light spectrum of far-off galaxies and stars is changing from the UV or optical range to the longer-wavelength infrared – and it is exactly this range that the Webb telescope is studying with its big mirror and optical instruments.
Looking Back at the Bang
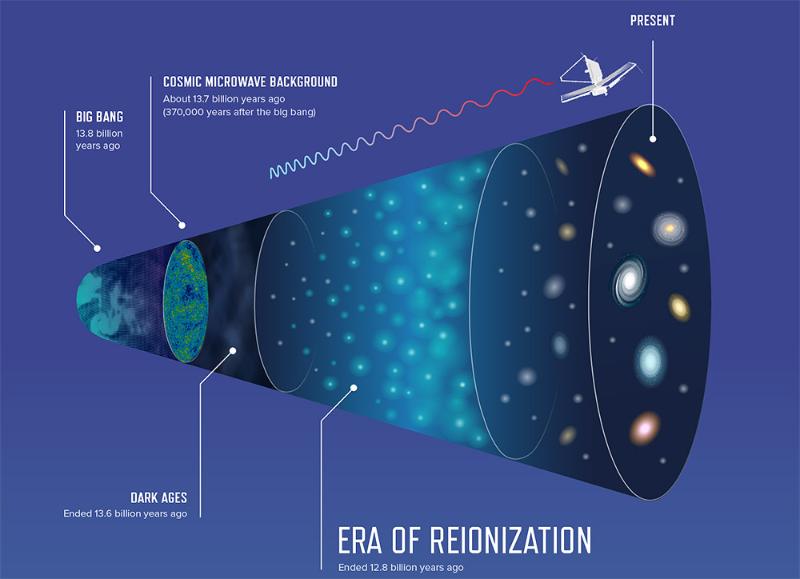
The earliest galaxies that have been discovered so far were created just over 500 million years after the Big Bang. However, it is still unknown when the first galaxies started to form. According to certain theories, there should be at least 50 galaxies that predate Hubble’s observation, although fewer are predicted by others. The Webb telescope is revealing for the first time when and how the very earliest stars and galaxies were created. This also provides light on how certain galaxies and quasars have managed to become so massively large at such a young age.
The James Webb Space Telescope can also discern the light spectrum of distant stars and galaxies with great resolution due to its high light output and precise optics. Its mid- and near-infrared operating spectrographs, MIRI and NIRSpec, are responsible for achieving this. The latter may be utilized to allow targeted radiation just from certain areas of the image since it contains almost 250,000 micro apertures. Scientists just open the spectrograph’s micro apertures to examine the spectrum of hundreds of these young galaxies for the first time.
Dark Matter, Galaxies, and Stars
The view into the infrared, however, has another benefit: Many cosmic events are encircled by thick dust clouds. The dust blocks the vision, making it impossible to see the creation of new stars and planets in visible light. On the other hand, these clouds are penetrated by infrared radiation with longer wavelengths. Thus, the JWST for the first time sheds light on the earliest phases of star formation and eventually the early history of our solar system.
Examining certain galaxies close up that are in the Milky Way’s local vicinity is another effort. Most of these dwarf galaxies are very dim, making it challenging to examine them closely with conventional telescopes. The Webb telescope’s sharp “eyes,” on the other hand, can keep track of how these galaxies and their stars move.
This might provide insight into how these galaxies interact with one another and how their mergers result in bigger star clusters.
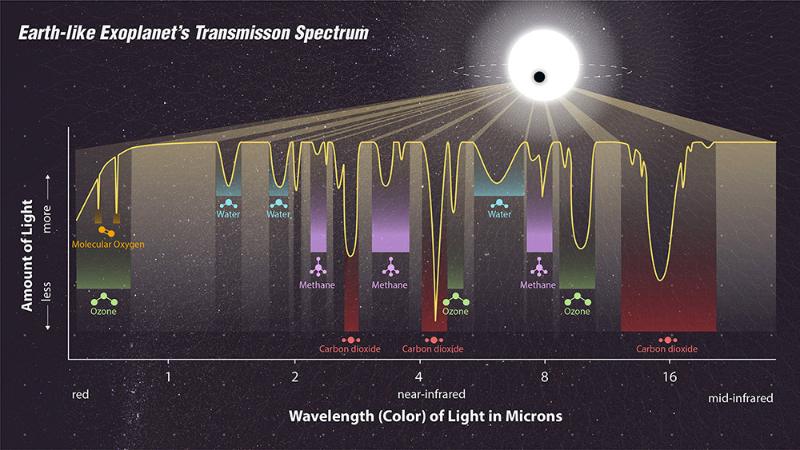
Thanks to the JWST, astronomers are now getting more knowledgeable about the distribution of dark matter and its function in galaxy formation. The characteristics of dark matter determine how structures develop in the cosmos. The JWST just needs to provide additional details about them. This will also help to explain why certain dwarf galaxies seem to have essentially no dark matter.
A Perspective on Alien Worlds
The James Webb telescope has great promise for planetary researchers as well. It can examine the atmospheres of exoplanets for the first time and look for molecular indicators of alien life. The NIRISS instrument, a near-infrared spectrograph that blocks the primary star’s outshining light, was created especially for this use. This enables James Webb to detect and examine the planet’s much softer light. Near Infrared Camera (NIRCam) detects light from the earliest stars and galaxies.
Additionally, Webb’s optics are sufficiently sharp to provide the first images of neighboring exoplanets. Several Earth-like exoplanets in our immediate cosmic neighborhood, such as the seven Earth-sized planets in the TRAPPIST-1 system, which is just 40 light-years away, are among the telescope’s first targets. That’s because a few of these planets could be suitable for supporting life.
The Webb Telescope’s Mirrors
James Webb’s main mirror is made up of 18 hexagonal parts and is the most remarkable component of the space telescope. It has a diameter of 21 ft (6.50 m) and a surface area of 269 sq ft (25 sq m), which is more than five times that of the Hubble mirror. This tiny optical apparatus needed some cutting-edge technology to be sent into space. Its use in the infrared range poses additional technical difficulties.
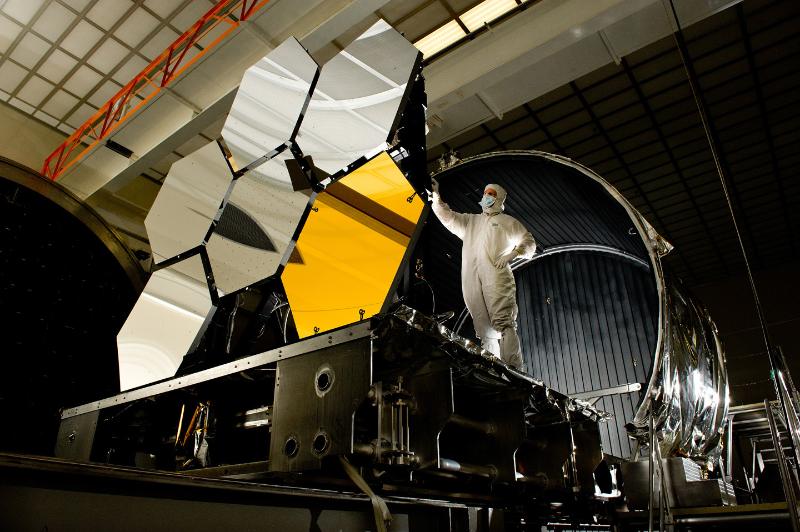
Weight is the first challenge for a space telescope with mirrors of this size since launch vehicle cargo is limited. All components must be as light as possible. The JWST would no longer be transportable if its mirrors were composed of glass with a metal coating, like those of the Hubble telescope. Because of this, NASA engineers decided to employ beryllium, a substance that has previously been used in supersonic planes and the space shuttle.
The Benefit of Beryllium
This alkaline earth metal is exceptionally stable despite being the second-lightest metal after lithium. Beryllium is very stiff compared to its bulk. Beryllium is hence even six times stiffer than steel. This honeycomb structure of millimeter-thick struts on the back of the mirror is enough to maintain the very thin mirror surface since it combines great strength and low weight.
Despite the JWST’s primary mirror being nearly three times bigger than the Hubble telescope’s, it weighs 1554 pounds (705 kilograms) instead of 1818 pounds (825 kilograms) due to the use of beryllium and the incredibly light design.
However, beryllium has another, arguably more significant benefit: it has very high thermal conductivity while also having a little reaction to temperature fluctuations. This is an essential characteristic of the mirrors’ curvature and almost nanometer-level alignment accuracy. At the low temperatures of space, beryllium maintains its stability across a broad range of temperatures, unlike other metals that expand when heated and contract again when cooled.
At these temperatures, beryllium is just amazing because even when the temperature changes, nothing moves at all. But beryllium can only be thermostable for a limited time at temperatures below minus 200 degrees C (-328 F). However, while the mirrors are being made in terrestrial temperatures, the alkaline earth metal also experiences a small amount of expansion. This required NASA engineers to calculate the mirror diameters to the nearest fractions of a micrometer, making them overly large on Earth but precisely the perfect size in space.
Careful Polishing
The first image of the Hubble Space Telescope around 30 years ago showed that even the finest material is useless if the optical surface does not have the proper shape and smoothness. The visuals were hazy back then due to a 0.16-inch (4 mm) difference in the mirror’s curvature. During a shuttle flight in 1993, spacewalking astronauts constructed and installed the new sensors and corrective optics on Hubble.
Polishing the beryllium segments of the JWST is also challenging since, unlike Hubble, it is located 932,000 mi (1.5 million km) away from Earth at Lagrange Point 2, making repair missions almost unfeasible. The most challenging and crucial technical step in the creation of the telescope was polishing the mirrors. The fact that the telescope’s mirror is made up of 18 segments that are polished independently yet must then properly complement one another further complicates issues.
The main mirror’s segments must be properly aligned to within one ten-thousandth of the thickness of a human hair for them to work as one big mirror. Each mirror has six actuators on the rear that allow for precise positioning in space. This adjustment, accurate to within ten nanometers, is only made once the mirror and the entire telescope optics have cooled down to the final working temperature of -220 to -243 degrees Celsius (-245 to -245 Fahrenheit).
Gold for the Best Light
The gold coating, however, is still a key component of the completed mirrors. This is because just this thin (about 100 nanometers) coating of precious metal makes it possible for the incoming infrared radiation to be nearly entirely reflected and focused on the secondary mirror. For this reason, the main mirror’s segments and the convex secondary mirror, which is located around 23 ft (7 m) above the primary mirror, both have gold coatings. This coating’s entire weight is just about 1.76 ounces or 50 grams, and a marble’s worth of gold might be contained inside it.
But how do you fit a sunshield the size of a tennis court and a telescope mirror the size of a 269 sq ft (25 sq m) payload capsule into a rocket? That was the precise issue that engineers working on the JWST mission had to address, and their solution was perhaps the most complex structure ever sent into space.
The Ariane 5 rocket’s payload capsule measures 15 ft (4.57 m) in diameter and 53.1 ft (16.19 m) in length internally. The major components of the space telescope had to be folded and dismantled like a sophisticated piece of origami in order for it to fit within this container, together with its enormous mirrors and protective shields. And everything that was so meticulously packaged on Earth must subsequently be unwrapped once again in space, automatically and at precisely the correct moment.
To do this, a vast army of levers, bolts, motors, gears, and wires was properly meshed. All the endeavors were in jeopardy if even one component failed. Space scientists always want to avoid in-orbit deployments at all costs. However, James Webb made it impossible to do that. Instead, the telescope had to carry out some of the most difficult tasks ever undertaken on an in-orbit deployment.
JWST’s Sunshield
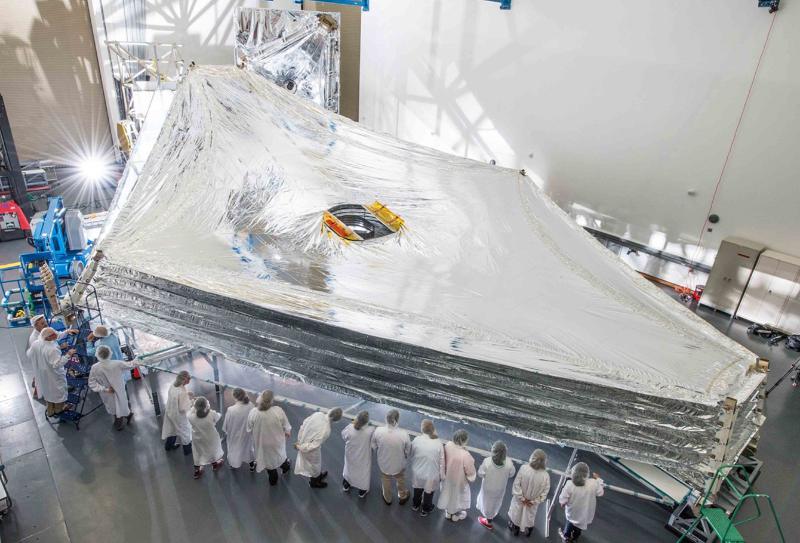
Similar to a parachute jump, the first challenge was to find the ideal amount of folding. Numerous engineers spent years experimenting with different ways to fold and compress the 0.025 to 0.05 millimeter thick, gossamer membranes of the tennis court-sized sunshield so they wouldn’t rupture during the launch of the rocket or while it was being deployed. The five-layer heat shield was finally arranged in such a way that it wraps two sides of the telescope.
The protective layer rolled back first and made room to unfold the five layers of solar protection in space, stretch them, and bring them into the proper kite-shaped configuration. The membrane holding mechanism must then be released by 107 retaining clips acting in the proper order. The membranes are then gradually stretched over a system of 90 hauling cables.
The automated packing and unpacking operation was tested several times using scaled-down replicas of the sunshield before being applied to the actual object. The sequence has to be further refined since membrane cracks and damage happened multiple times. In the end, the deployment process of James Webb was sluggish yet precisely and orderly timed.
In vacuum chambers, weightlessness can be replicated, but space’s vacuum cannot. The team consequently tested the automated unfolding from several angles to at least change the gravity impact. However, there was no dress rehearsal while the telescope was suspended in space.
The Unfolding of the Mirror
The opening of the main mirror was equally important in the deployment of the James Webb Space Telescope. The telescope’s 18 hexagonal parts had to be joined and aligned to within a few hundred nanometers for the Webb to function properly. Two of the mirror’s edges, each made up of three segments, were folded in for transportation since the complete mirror, with a diameter of 21.3 ft (6.5 m) was too enormous to fit within the Ariane rocket. It was taking over three hours to unfold these “wings” again.
Similarly important was the structure’s unfolding, from which the 74-centimeter secondary mirror hung out. The three 25 ft (7.60 m) rods were folded up and laid parallel to the main mirror for transit. Then the secondary mirror had to move into the path of the light focused by the first mirror. Only after these first procedures can the mirrors’ delicate adjustment via the actuators on their backs start. When all 138 actuators function properly, the direction and curvature of each mirror can be controlled to provide an ideal image.
With 178 processes involved, the Webb observatory performed 50 crucial unfolding steps during its deployment. The Webb telescope deployment is by far the trickiest task any spacecraft has ever had to do. However, nothing about Webb is easy—and nothing has ever been done before.
How Did All Happen?
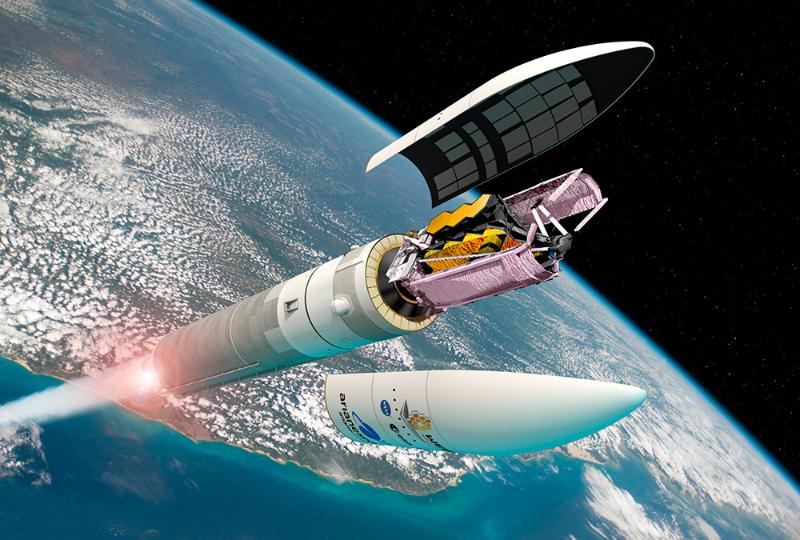
Every time NASA rovers arrive on Mars, the difficult, multi-step deployment entails “seven minutes of anxiety.” However, people engaged with the James Webb telescope had to go through 29 days of anxiety all at once. The time required to fully deploy and position the space telescope’s major components was that amount of time.
Just a few minutes after launch, the crucial step started: The two halves of the payload capsule were jettisoned, and 28 minutes later, the telescope was freed from the Ariane 5 launch vehicle’s burned-out upper stage. From this point on, the Webb telescope had to navigate to Lagrange Point 2 on its own. It initially extended its solar sails to provide itself with energy before doing this. The base station’s communication antennae came up around two hours after launch.
Twelve hours after launch, the JWST completed its first crucial action: the propulsion jets activated, providing the telescope with the required power and guiding it in the appropriate direction for the journey to the Lagrange point. The Webb telescope entered lunar orbit in only two days, traveling far more quickly than the Apollo capsule from more than 50 years ago.
The Deployment Took Two Weeks
The first significant deployment started in three days: To provide the telescope with the required separation from the sunshield and the other “warm” areas of the observatory, the mounts for the sunshield first folded out. After that, the entire optical complex of the telescope lifted by around 6.5 ft (2 m). The sunshield’s five layers then progressively spread out over the course of the next seven days.
The mirror components took up the second week of the unfolding phase. The secondary mirror with its telescoping poles was set up on the tenth day, and the main mirror’s sides folded in on the twelfth. It was essential for these structures to be correctly activated at this time. The icing was a thing to watch out for as it must not develop on the optical components. Other than that, if the water vapor and other gases were to condense on the cool mirror surfaces, the probe’s still-warm components would be at the risk of outgassing (being subjected to the vacuum of space).
Calculate and Hope For the Best
The most dangerous and error-prone aspect of the operation was over after 29 days. Since there are no live cameras on the James Webb telescope to monitor its overall standing, the ground staff was unable to see the procedure if anything went wrong. But the numerous sensors that offer feedback everywhere throughout the intricate structure as to whether a component has been released, extended, engaged, or otherwise performed its role are the main sources of information on the James Webb.
The engineers have to come up with a workable solution if one of these sensors reports an error using the observatory’s onboard resources. For instance, the JWST could attempt to shake a trapped bolt, clamp, or cable free. The scientists jerk the whole spaceship back and forth to do that.
The James Webb Telescope carried out one more flying maneuver to complete the deployment phase and reach its orbit around Lagrange Point 2. Everything went as planned, and the biggest and most complicated space telescope ever launched into space was able to start operating. After that, scientists adjusted the mirrors, checked all the instruments, and calibrated them for many months before the scientific expedition began. The James Webb Space Telescope is answering the fundamental questions of where we came from and why life originated on Earth out of all places.
Bibliography
- NASA JWST – “How big is Webb?“
- “JWST Orbit”. JWST User Documentation.
- “Will Webb be able to tell us more about the Big Bang?” – NASA JWST