Nuclear fission is the splitting of a heavy atomic nucleus into two medium-heavy atomic nuclei by bombardment with neutrons. In this process, neutrons are released and energy is given off which is known as nuclear energy. Nuclear fission is a special form of nuclear transformation. It was discovered in 1938 by Otto Hahn, Fritz Strassmann, and Lise Meitner.
Nuclear fission is the conversion of an atomic nucleus into new nuclei. An example of such nuclear fission is when neutrons hit uranium-235, a nuclear transformation occurs into uranium-236, which decays into two nuclei in fractions of a second. However, there are multiple possibilities. A uranium nucleus can also decay into other nuclei, such as lanthanum and bromine, selenium and cesium, or antimony and niobium. In total, more than 200 decay products of uranium are known.
Each nuclear fission releases 2 or 3 neutrons. In general: By bombardment with slow neutrons, heavy atomic nucleus (like uranium, and plutonium) can be split into medium-heavy atomic nuclei which release a tremendous amount of energy.
Why Does Nuclear Fission Release Energy?
Nuclear fission releases energy because the fission reaction converts mass into energy. There is no chemical reaction taking place in nuclear fission unlike in the combustion of fossil fuels. Therefore no CO2 is released into the atmosphere.
In nuclear fission, the mass of the initial nucleus plus the absorbed neutron is greater than the mass of the nuclei being created, including the neutrons being released. In fission, a mass defect occurs. According to the E=mc2 discovered by Albert Einstein in 1905, the reduction of mass corresponds to released energy.
When a uranium nucleus fissions, an energy of about 3 x 10-11 Joule is released. This may seem little. However, this refers to one nuclear decay of an atom. There are 2.6 x 1024 amount of atoms in Uranium-235. If we consider the number of atomic nuclei contained in a kilogram of uranium and assume that they all decay, the energy released from the fission of uranium is then 8.6 x 1012 J. This is about 640.000 times the energy released when 1 pound of hard coal is burned (290,000 times for 1 kg).
The Chain Reaction in Fission
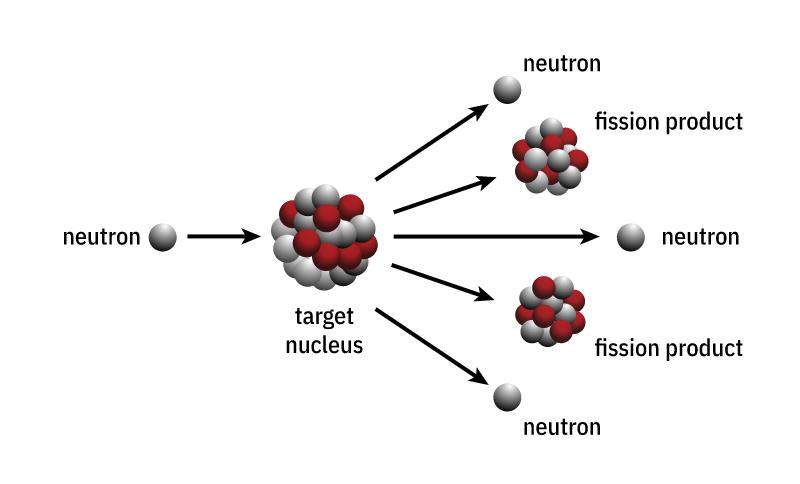
If the neutrons released during nuclear fission meet other fissile material with the “right” speed, they can cause further nuclear fissions. The result is a reaction that continues by itself called a chain reaction. If this chain reaction is not moderated, it is called an uncontrolled chain reaction (nuclear weapons). Uncontrolled chain reactions occur in atomic bombs.
However, certain materials can be used to limit the number of neutrons to moderate the chain reaction. Such a moderated chain reaction is called a controlled (nuclear power) chain reaction. Controlled chain reactions take place in nuclear reactors of nuclear power plants. The enormous amounts of energy released during nuclear fission make nuclear power a genuine energy source.
The Energy Efficiency of Nuclear Fission
The process of nuclear fission is very efficient. When 1 kilogram (2.2 pounds) of U-235 is fissioned, only about one gram of mass is lost (one part in a thousand), which is converted into heat energy. Applying Einstein’s relationship E=mc2, this gives a value of about 25 million kilowatt-hours. This corresponds to combustion energy of about 5,500,000 pounds (2,500,000 kilograms) of hard coal with an energy content of 3200 kilocalories per pound (7000 kilocalories per kilogram). The energy yield per pound of fuel in nuclear fission is about 5.5 million times higher than that of burning hard coal (2.5 million times per kilogram).
The reason for these enormous efficiency differences lies in the usage of two natural forces with different degrees of interaction. In combustion, the underlying chemical processes take place in the electron shell of atoms which results in electromagnetic interaction. In nuclear energy, there is a much larger strong interaction that binds the nucleons together. Therefore, the nuclei of the atoms play a decisive role in the resulting nuclear energy.
The decisive factor here is the magnitude of the binding energy per nucleon in the nucleus. It is not constant for the elements, but increases from the lightest element, hydrogen, at first very steeply and then more slowly up to the heavier elements such as krypton. After that, it drops slightly to the heavy elements. When heavy nuclei split into two medium-heavy ones, the difference in binding energies is released in the form of heat by the motion of the fission products.
The difference in the strength of the interactions is also expressed in another figure: The decomposition of a heavy atomic nucleus into two moderately heavy nuclei results in an amount of energy about 400,000 times greater than in chemical reactions between whole atoms. These enormous differences may explain why nuclear energy is highly attractive in terms of energy economics, but on the other hand, because of the enormous energy density that must be controlled, it requires a particularly high degree of responsibility and care concerning the safety of nuclear power plants.
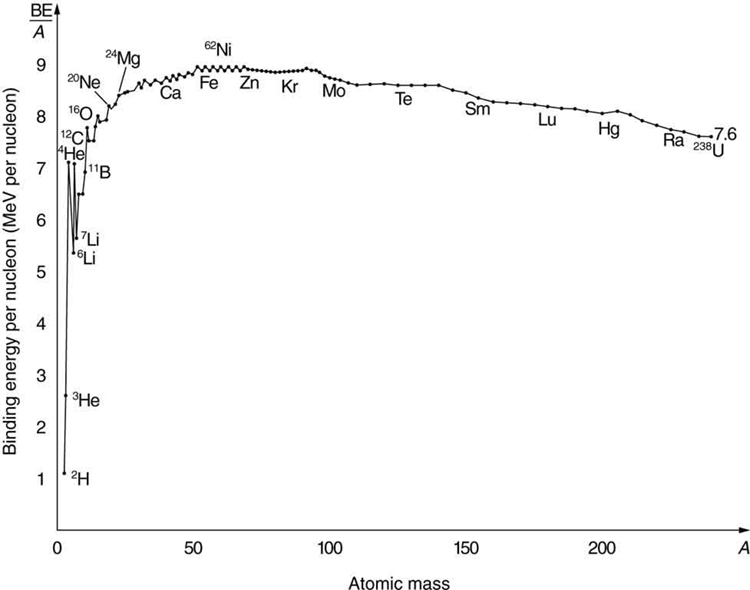
The binding energy curve graph above shows the dependence of the binding energies per atomic nucleus as a function of mass: for hydrogen, it is just over one megaelectronvolt. Then the curve rises steeply as the size of the atomic nucleus increases; for helium, there is a peak of 7 megaelectronvolts, and for lithium is 5.5. The rise continues through oxygen, which has 8 megaelectronvolts. For iron, the maximum is just under 9. There, the curve falls flat until uranium, which has 7.5 megaelectronvolts of binding energy.
The Discovery of Nuclear Fission
In the 1930s, many physicists and chemists were interested in knowing more about radioactive radiations.
The Italian physicist Enrico Fermi bombarded numerous elements with neutrons and found that almost all substances on Earth could be transformed in this way. He called the newly formed substances transuranium elements as he first assumed that all these substances were beyond uranium in the periodic table of the elements, which means they had an atomic number of more than 92.
Later both Ernest Rutherford in England and the Joliot-Curie couple in France also experimented with nuclear transformations. The daughter of Marie Curie, Irene Joliot-Curie and her husband Frédéric Joliot-Curie discovered artificial radioactivity in 1934.
A few years later in Germany, the chemist Otto Hahn, the physicist Lise Meitner, and Fritz Strassmann at the Institute of Chemistry in Berlin-Dahlem were among the people who worked together on transuranic. Lise Meitner had to emigrate from Germany in 1938. Hahn and Strassmann irradiated uranium with neutrons and studied the resulting nuclides, which were present only in minute quantities. In the process, they made a discovery in December 1938 that seemed improbable even to them.
Reluctant to publish their ‘strange results’ as ‘nuclear chemists’, they concluded that their radium isotopes had the properties of barium which means that the new bodies were not radium but barium which contradicted all previous knowledge in nuclear physics.
Those new fission products were unambiguously identified a short time later. Bombardment of uranium with neutrons produced krypton and barium. At the same time, each nuclear fission released three neutrons and free energy. A short time later, further fission products of uranium were detected. Thus nuclear fission was discovered, for which Otto Hahn (1879-1968) received the Nobel Prize in Chemistry for the year 1944 in 1945, after the end of World War II.
The plain possibility of generating energy from nuclear fission had been debated in 1939 and was known by leading nuclear physicists of the time. What was also known, however, was the great technical effort required for it to occur. With the beginning of World War II, the question of whether nuclear energy could also be used for military purposes increasingly came to the fore. In 1942, intensive work on nuclear weapons began in the USA. The first nuclear weapon explosion, an experimental bomb, took place on July 16, 1945, in the desert of New Mexico (USA). On August 6 and 9, 1945, the first US atomic bombs exploded over the Japanese cities of Hiroshima and Nagasaki, killing hundreds of thousands of people.
Relation Between Fission and Structure of Atomic Nuclei
The atomic nuclei of the chemical elements are composed of two different particles: The electrically positively charged protons and the electrically neutral neutrons. Both are also called nucleons. They have almost the same mass and are held together by the so-called nuclear force, which physicists also call the strong force. It is by far the strongest of the four forces occurring in nature: gravitational, electromagnetic, weak, and strong force. Unlike the electromagnetic force, the nuclear force acts only in an attractive manner that applies only to its nearest neighbors.
The positively charged protons repel each other in the atomic nucleus because of their equal electric charge. As long as this electrical repulsion, called Coulomb force is compensated by the much stronger nuclear forces, the nucleus remains stable or does not become radioactive. The nuclear forces of the electrically neutral neutrons also help in this process.
The amount of the protons determines which element will be used in nuclear fission. The higher the number of protons and the heavier the elements become, the more neutrons are needed for the fission- up to a significant surplus compared to the protons – to compensate for the repulsive Coulomb forces. These forces can become very large because of the very small distances between the protons since the Coulomb forces are inversely proportional to the square of the distances. However, at high proton numbers, as in uranium-92 which is at the edge of the balance between Coulomb repulsion and binding nuclear forces, the nuclei start to become unstable and thus radioactive.
But even with the elements with a smaller number of protons nuclei can still become unstable. In general, only the interaction between the Coulomb repulsion of the protons and the attractive strong nuclear forces between all particles is decisive. This is due to the long range of the Coulomb force causing all the protons to interact with each other. The repulsion force grows quadratically with the number of protons. Because of its shorter range, the attractive force of the strong interaction acts only with the nearest neighbors and grows only linearly with the number of nucleons. Depending on the ratio of the number of protons and nucleons, the repulsion prevails and the nucleus becomes unstable.
What Is the Significance of Uranium-235 for Fission?
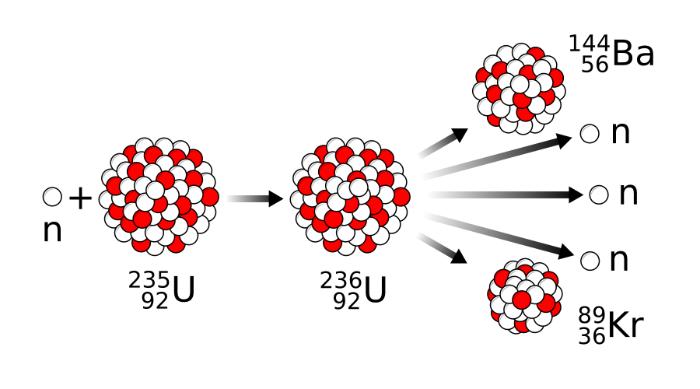
If one of the same element has an atomic nuclei with different numbers of neutrons, they are called isotopes. For the use of nuclear energy for power generation, the uranium isotope 235 is quite important. It contains 235 nucleons, of which 92 are protons and 143 neutrons. In nature, it occurs in a proportion of only 0.7 percent. The most abundant uranium with 99 percent occurrence is the isotope U-238 with 146 neutrons. With a tiny portion of 0.005 percent also exists the isotope U-234 with 142 neutrons.
What makes U-235 special is that it splits into two lighter atomic nuclei (fission products) as soon as another neutron is added to it. An intermediate nucleus of uranium is formed first, namely the isotope U-236, which is in an highly excited state. The excitation energy corresponds to the binding energy (while the kinetic energy of the neutron can be neglected) released by the neutron capture reaction. The energy is relatively high due to the magnitude of the strong interaction.
U-236 is unstable and therefore releases its excitation energy for at least 10 seconds mainly by fission to the two medium-heavy nuclei. These fission products are positively charged. Therefore, they repel each other due to the Coulomb force and are accelerated to full velocity for up to 20 seconds. Their kinetic energy, which is converted into heat, accounts for less than ten percent of the energy released during nuclear fission. Up to twenty percent of fission energy is in the radioactivity of the newly formed medium-weight nuclei.
The Cause of Radioactivity in Fission
The nuclei of the fission products are not always the same. They have statistically different charge numbers and thus, belong to different chemical elements. When the number of elements formed during fission is compared with their charge numbers, a saddle-shaped curve with two maximum is obtained. In the first maximum, there could be elements like strontium, krypton, or yttrium, and for the second maximum xenon, cesium, or barium. Most of these fission products are radioactive due to an excess of neutrons and only turn into stable end products after long decay series. In total, about 200 different fission products are known today.
In addition to the two fission nuclei, 2 to 3 neutrons are also produced, which can be used to fission other U-235 nuclei and release further energy and neutrons. This is called a chain reaction. It is crucial for maintaining the fission process and the use of nuclear energy.
The probability of a neutron attaching itself to U-235 depends on its speed. The probability increases with a lower speed. Since the neutrons released during fission are too fast to attach, they must be slowed down to so-called thermal speeds by collision processes with the atomic nuclei of a moderator. To make the collisions effective, the atomic nuclei of the moderator should have as much mass as possible as the neutron. Water is a suitable moderator in nuclear fission as the nuclei of the two hydrogen atoms in the H2O molecule consist of protons and have practically the same mass as the neutrons to be decelerated.
Structure of the Nuclear Reactors
In a nuclear reactor, uranium, and moderator are arranged in such a way that allows a continuous series of fission reactions. When this is maintained with control devices, the fission reactions create nuclear energy as heat. The ratio of the two uranium isotopes 235 and 238 is a critical variable. In the light-water reactors operated predominantly around the world today, the 0.7 percent uranium-235 in the natural uranium is not sufficient to maintain a continuous series of fissions. Uranium-235 must be enriched to about three percent before it is used in reactors that require particular uranium enrichment facilities.
The neutrons released do not only fission the uranium-235 but they can also be captured by the ruling uranium-238. This creates the so-called transuranium elements such as the new isotope plutonium-239 created by the radioactive decay which is a fissile material and can also release more energy. Depending on the type of nuclear reactor, it can contribute about 30 percent to the energy generated by the fission process. Transuranic elements also include chemical elements such as neptunium, americium, and curium.
The transuranic elements contain isotopes, some of whose radioactivity is very long-lived. This allows the total radioactivity in the spent nuclear fuel assemblies to largely decay only after several hundred thousand years. Overall, after about three years of operation (pressurized water reactor types), the spent fuel elements of the light water reactors contain close to one percent uranium-235, half a percent uranium-236, 95 percent uranium-238, and close to one percent plutonium isotopes. The remainder is made up of fission products (three percent) and other transuranic (less than 0.05 percent), the so-called actinides. In the end, the percentage of U-235 is almost equal to the percentage of natural uranium.
The concept of the closed fuel cycle nuclear reactors aims to chemically separate the uranium and plutonium by reprocessing the spent fuel elements to recover them for energy production, as well as to safely store the radioactive residue for a very long time. This will allow about 97 percent of the spent fuel to be reused. More advanced nuclear reactor concepts include additional separation of the long-lived radioactive materials, by interaction with fast neutrons, to convert them into much shorter-lived chemical elements for final storage, also called transmutation.
The open fuel cycle reactors allow storing the spent fuel elements and other highly radioactive waste from nuclear power plants directly. Hundreds of tons of spent fuel are generated each year by the countries with nuclear power plants that can fill several Olympic pools. Besides uranium, the chemical element thorium is the second most important element for the production of nuclear energy.
Sources:
- P. K. Kuroda (1956). “On the Nuclear Physical Stability of the Uranium Minerals” (PDF). The Journal of Chemical Physics. 25 (4): 781. Bibcode:1956JChPh..25..781K. doi:10.1063/1.1743058.
- Bulgac, Aurel; Jin, Shi; Stetcu, Ionel (2020). “Nuclear Fission Dynamics: Past, Present, Needs, and Future”. Frontiers in Physics. 8: 63. arXiv:1912.00287. Bibcode:2020FrP…..8…63B. doi:10.3389/fphy.2020.00063.
- V, Kopeikin; L, Mikaelyan and; V, Sinev (2004). “Reactor as a Source of Antineutrinos: Thermal Fission Energy”. Physics of Atomic Nuclei. 67 (10): 1892. arXiv:hep-ph/0410100. Bibcode:2004PAN….67.1892K. doi:10.1134/1.1811196. S2CID 18521811.