The history of the periodic table marks a search for the order of the elements, the fundamental components of all matter, and the building blocks of our existence. Dmitri Mendeleev, who hails from the farthest reaches of Siberia, changed the world with a simple table. What Dmitri Mendeleev proposed in 1869 contained the order of the elements, which is the foundation of the universe. The question of whether there was a fundamental order or regularity underlying the elements was first posed by ancient scholars. They attempted to discern order among the elements after realizing that some substances resembled one another more than others.
But Dmitri Mendeleev and his periodic table of the elements, which others further developed several years later, represented the critical advance. Along with organizing the known elements according to their atomic weights and formative properties, Mendeleev also identified the underlying regularities.
While entire worldviews have crumbled in physics and biology over the past few decades, the Russian chemist’s Periodic Table has stood the test of time. Whether it be nuclear fission, the discovery of new elemental groups, or the understanding of the atomic structure, all advancements in chemistry and atomic physics have only served to confirm and extend Mendeleev’s brilliant design. But what exactly was his discovery’s secret?
What Is An Element?
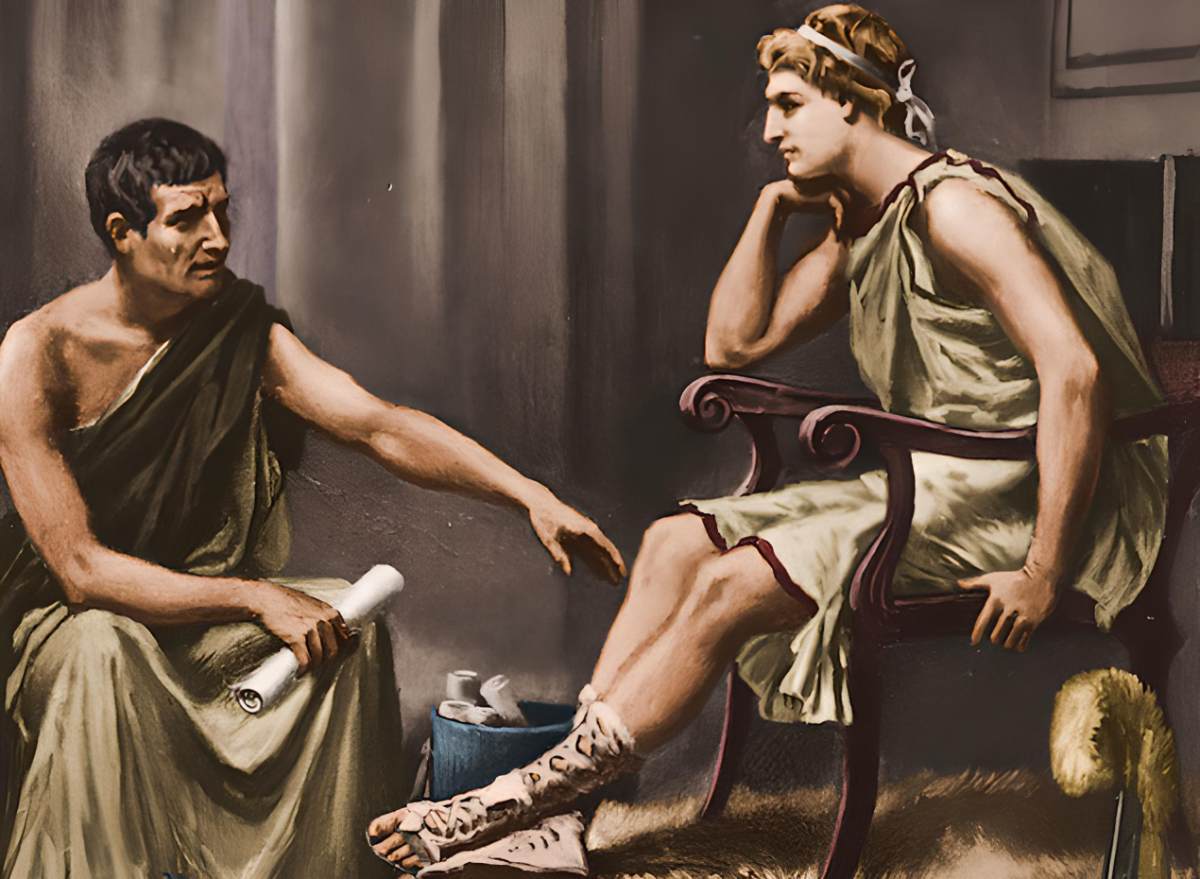
Finding the Primordial Substance
What is the origin of all things? Why is metal hard and water liquid? Why do coal and sulfur behave differently than copper and silver? These are by no means modern-era issues; ancient people were already troubled by them. They, too, ceased to simply take the natural world for granted and yearned to comprehend its fundamentals.
Early Greek naturalists and philosophers agreed that there must be some kind of order or unifying principle at work in the universe. Maybe there is a primordial material that explains how different substances behave and how they have certain properties. The solution was obvious to the Greek philosopher Thales of Miletus in the year 600 BC: water. He thinks that everything on the Earth’s disk is just a different aspect of this primordial substance. Today we know that when heated sufficiently, even solid, hard metals can change into a liquid state. At most, it was debated whether fire or air—as Heraclitus postulated—was the enigmatic primordial substance.
The Four Elements of Aristotle
Aristotle unified these theories into his four-element doctrine about 300 years later. He gave each “element” characteristics there; for instance, the earth is cold-dry and the water is cold-moist. Because all matter was a combination of its original constituent parts, a substance’s properties and behaviors depend on the ratio of those parts. But above all, in Aristotle’s view, there was still the quintessence, the fifth element, the ether, which gave life to everything else and was the source of all existence.
What Aristotle did not know at the time was that his ideas would influence the Western worldview for almost 2,000 years. The four fundamental elements were referred to by everyone from the Greeks and Romans to the alchemists and doctors of the Middle Ages and the Renaissance.
Robert Boyle: The Irish Rebel
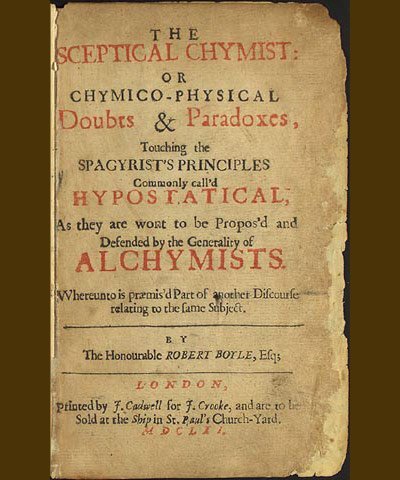
A young Irishman named Robert Boyle, the youngest son of the Earl of Cork, announced the sudden end to this age and the start of modern chemistry in the 17th century. Boyle spent some time in the Kingdom when he was twelve years old, as was customary for aristocrats at the time. He visited France, Switzerland, and Italy and studied under the greatest scholars of the day while also making friends with many quacks and alchemists. In 1644, he returned to Ireland and started his own experiments, boiling, evaporating, and distilling while examining the objects under a microscope. He was particularly intrigued by the behavior of gases.
Boyle began to question the four-element theories of Aristotle and Paracelsus more and more as they were based on conjecture rather than actual scientific research. Boyle observed that whether substances combine or just mix does make a difference and that many substances can be broken down to a very great extent, but some cannot. One such substance was phosphorus, which the pharmacist and alchemist Hennig Brand discovered a few years earlier. This substance, which was extracted from urine by evaporation and annealing, appeared to be incredibly stable, resisting being broken down further.
In 1661, Boyle was finally certain that there must be more than four elements. All substances must also be elementary if they cannot undergo further chemical reactions. The Irish scientist and natural philosopher ultimately put an end to the age of alchemy with his new concept, which he published in his book “The Sceptical Chymist”; modern chemistry had arrived.
Karlsruhe Congress Lit the First Spark
The First International Congress of Chemistry
Karlsruhe, September 3, 1860: The second meeting room is humming with activity. 140 men shake hands, talk, and gesticulate while most are dressed in festive dark suits. Then it finally begins. “As provisional chairman, I have the honor of opening a congress that is unprecedented, that has never existed before. For the first time the representatives of a single and indeed the newest natural science have gathered here,” says Karl Weltzien, chemist and meeting organizer at the Polytechnic School in Karlsruhe.
The first-ever International Chemical Congress, which went down in history, was officially opened with these words. Because Karlsruhe Congress served as a catalyst for some of the most important developments in contemporary chemistry, including the Periodic Table. In addition to well-known researchers like Robert Bunsen, Dmitri Mendeleev, August Kekulé, Jean-Baptiste Dumas, Carl Fresenius, and Louis Pasteur, the congress brought together the brightest minds in this young field.
Atomic Weight Disarray
However, there was still a great deal of bickering, debate, and fighting going on. Because the congress was about nothing less than the basis of all things: the atoms. Or more precisely, the atomic weights.
Atoms were already universally acknowledged to be the fundamental units of matter. However, there were six different ways to state an atom’s weight. While some considered hydrogen to be the standard for all things and assigned it the atomic weight 1, others viewed oxygen as the supreme force and based all other atomic weights on it. But not only did this lead to uncertainty, but it also made it challenging to determine whether an analyzed substance was an element or not.
A young Russian chemist named Dmitri Mendeleev was one of the congress attendees. Mendeleev was able to attend the prestigious university in Saint Petersburg despite being from the remotest Russian province, the town of Tobolsk east of the Urals. He had even been sent on a two-year study tour to France and Germany after receiving his Master’s degree. Mendeleev focused primarily on physical chemistry while pursuing his doctorate in the Heidelberg laboratories of Robert Bunsen and later of Gustav Kirchhoff. His research included measuring the molecular weights and the precise volume of liquids.
An Italian Solution
The speech by the Italian chemist Stanislao Cannizzaro was given on the third and final day of the congress. He suggested a solution based on Amadeo Avogadro’s gas law to address the problem of atomic and molecular weights. According to the latter theory, an ideal gas should always have the same number of particles at the same volume, pressure, and temperature. Avogadro used the term “particles” in this context to refer to diatomic molecules. Cannizzaro said in his speech, “The vapor density therefore provides us with a means of unequivocally determining the weight of molecules of different substances, whether atomic or as a compound.”
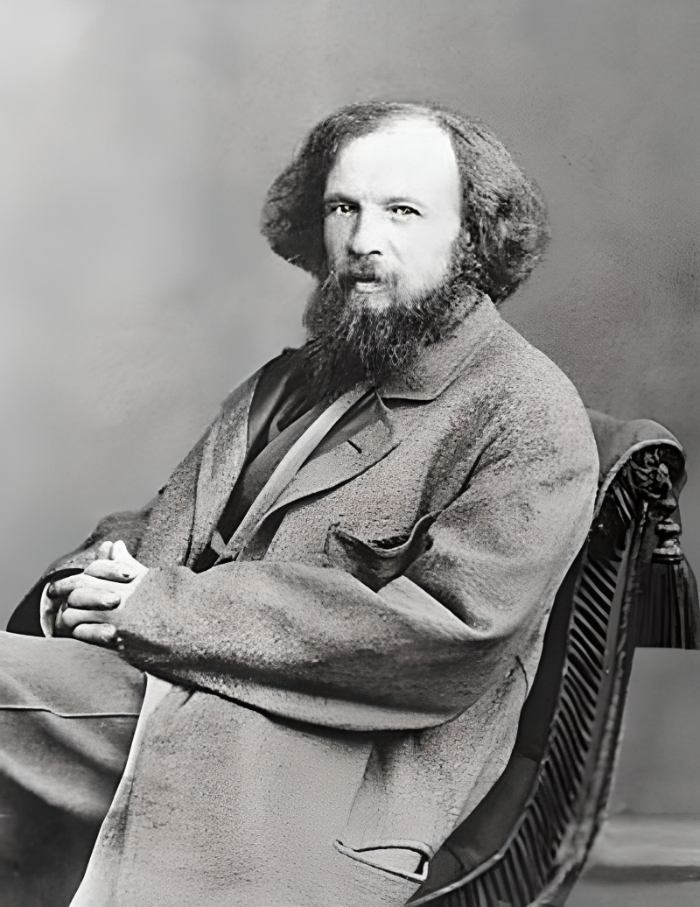
When Cannizzaro made this proposal, Mendeleev, who was only 26 years old, was also present in the room. In the form of a periodic table, this talk was a starting point for Mendeleev. After all, he had been preoccupied with the issue of how to categorize the roughly 60 known elements for a number of years. Mendeleev later stated in one of his writings that “It is one of the functions of science to discover the existence of a general principle of order in nature and to find the reasons that govern this order. The cathedral of science requires not only material, but a design, a harmony.”
Mendeleev was not alone in holding this opinion; the congress in Karlsruhe, which led to the correction and unification of many atomic weights, set off a veritable race to see who could explain the properties and behavior of the elements first.
Mysterious Similarity Between Various Elements
Even before the 1860 Karlsruhe Congress, many chemists had noted that while all elements were the same, some were more similar than others. This suggested that some elements could be related in some way. For instance, all three of the following elements—chlorine, bromine, and iodine—are gaseous or barely volatile, intensely colored, and highly reactive with metals and hydrogen. However, some substances, like lithium, sodium, and potassium, are solid and have a strong reaction with water.
Even among these related groups, however, there are clear distinctions: Lithium moves leisurely across the surface of the water, reacting with it and giving off hydrogen until it disappears. A lump of sodium, however, moves across the surface with a furious hoist, but catches no fire. Potassium, on the other hand, catches fire the moment it touches the water, burning with a pale purple flame and spraying globules of itself everywhere.
The Law of Triads
Johann Wolfgang Döbereiner, a German chemist, also noticed these similarities in 1829. When he placed the related elements calcium, strontium, and barium next to one another, the atomic weight of strontium was exactly equal to the average of the other two, indicating that atomic weights appeared to be a factor in these affinities. Lithium, sodium, and potassium created another trio that shared this property. After all, Döbereiner’s “Law of Triads” allowed for the grouping of 30 of the 53 elements that were known to exist at the time. The other elements, such as the gases oxygen, hydrogen, and nitrogen, didn’t want to adhere to his theory. And his contemporaries did as well. They found the entire situation to be “insufficiently conclusive.”
The Law of Octaves
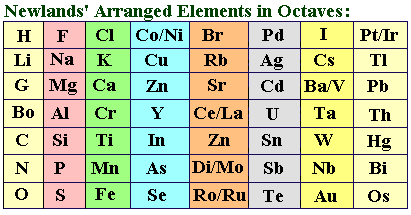
35 years later, the English chemist John Reina Newlands attempted an elemental sorting as well, bolstered by the “tidied up” atomic weights in Karlsruhe. Newlands started by listing the known elements in ascending order of atomic mass. He quickly noticed that the properties of the elements in this order followed a pattern: the fundamental characteristics of the seven earlier substances repeated after every eighth element. This made John Newlands think of music and the octave principle, which states that the same note can be repeated with a different pitch. He called his discovery the “Law of Octaves” as a result.
Newlands reorganized his series and now arranged the elements in columns with seven rows each to make the parallels even more obvious. There were now a startlingly large number of comparable elements in the table’s rows. In this way, magnesium was next to calcium, strontium, and barium, while fluorine was next to chlorine, bromine, and iodine.
There were also significant outlier elements in between, such as silver in the row between lithium and sodium, or nickel and palladium, which he placed between chlorine and iodine. But this cannot be possible today because of how different their elemental properties are from those of their neighbors. Other series, especially the lowest, were made up entirely of disparate elements with no indication of a system or even of similarities.
Yet why? Newlands believed he was getting close to finally ordering the elements and that his system could not be wholly incorrect. But he was unable to control the “outliers” problem.
But there was also someone else who was also obstinately working toward this problem’s solution thousands of miles to the east: Dmitri Mendeleev.
Mendeleev’s Periodic Law
“It’s all formed in my head”
Early 1869 in Saint Petersburg. Dmitri Mendeleev, who was currently a professor of pure chemistry at the University of Saint Petersburg, had been sequestered in his office for several weeks. Other than his lectures, he didn’t have any free time. Not even for his hairdresser, not for his wife and two kids, not for evening outings to the ballet or the theater. He was searching for something. Like many of his contemporaries, he was searching for the fundamental arrangement of the elements, and he felt as though he was getting close.
Ability of an Element to Bond
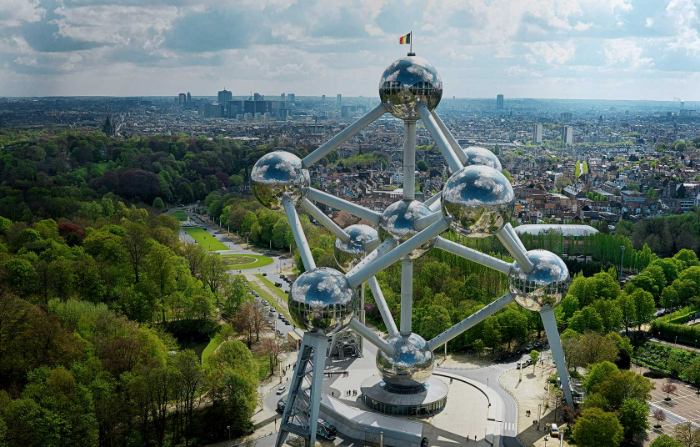
Mendeleev had long understood that there must be a link between atomic weights and the characteristics of chemical elements, just like John Newlands. But in contrast to the latter, he also considered valence or value. This characteristic of elements, identified by Edward Frankland in 1852, showed how strong was their ability to form bonds with other atoms, such as hydrogen atoms. Or, to put it another way, the number of “free arms” available for bonding between atoms.
Like Newlands, Mendeleev first arranged the elements in ascending order of atomic mass. He then turned his attention to the valences, noting that these too appeared to occur in cycles. From lithium to fluorine, they rose from one to seven, but sodium then started at one, and the valences rose once more to chlorine. Then, however, the scheme of seven came to an end: the following period was already significantly longer; it appeared that at this point, the elemental behavior had departed from Newland’s rigid octave scheme.
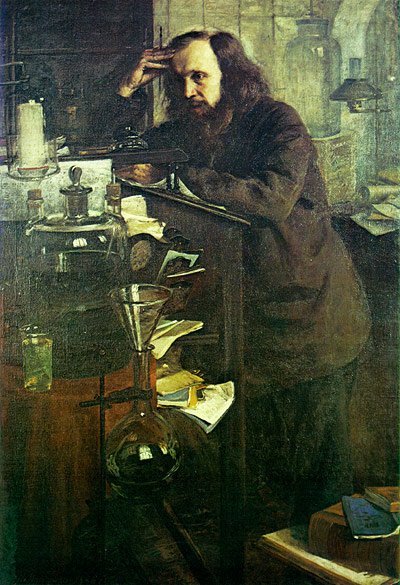
Elemental Card Game
Which scheme, then, did the elements fit into? When a friend paid Mendeleev a visit in his office at the university in February 1869, this was the exact question that Mendeleev was still struggling with. The friend asked him in horror what he was working on as he turned to face the hollow-cheeked, red-eyed figure with completely wild hair and a beard. Mendeleev explained that although he had found that there was a periodic system of elements, he was unable to create a matching table system or a law. The worn-out chemist laments, “It’s all formed in my head, but I can’t express it.”
Mendeleev, however, was persistent. In a later statement, he said, “When you’re looking for something – be it mushrooms or some kind of law – there’s no other way than to keep looking and trying again.” His primary tool for these experiments was a stack of plain cardboard cards rather than a Bunsen burner or any other chemical apparatus. He noted an element’s atomic mass and its most distinctive properties, such as valences, on each of them. He then shuffled the cards on his table until a system was finally formed.
The Seven Groups
Everything suddenly appeared to be straightforward: the elements were divided into seven groups, each of which combined elements with related properties perpendicular to one another. The atomic masses and valences of the elements rose in the rows of the system’s structure, or its periods, as a result of the placement of these groups next to one another. The group of metals from the alkaline earth came first, then the alkali metals. The “earth metals,” led by boron, were then followed by the carbon, nitrogen, and oxygen groups. The conclusion was formed by the halogens, which included fluorine, chlorine, bromine, and iodine.
Mendeleev now understood that this was the only way the elemental order could function fundamentally. However, at first glance, he too appeared to have some anomalies that disturbed the overall picture.
What Made Mendeleev’s Periodic Table Unique?
German chemist Lothar Meyer also worked on a nearly identical periodic table almost simultaneously with Mendeleev. Although Meyer also understood the fundamental concept of groups and periods, he could only come up with six groups. Meyer also encountered a few factors that significantly disrupted the overall picture, similar to Mendeleev.
How Should Errors and Gaps Be Handled?
For instance, beryllium, which was thought to be the third lightest element, belonged up front. However, it couldn’t fit into the plan because the first group was made up of alkali metals, which beryllium could be a part of. Tellurium, an element with an atomic weight of 127.6 and a valence of 2, also appeared to be positioned incorrectly. According to its atomic weight, the element should be behind iodine, but its valence clearly corresponded to that of the oxygen group, placing it in the element group prior to iodine and the other halogens.
Mendeleev, in contrast to Meyer, had no hesitation whatsoever. Because he was so confident in his theory, he could only conclude that the atomic weights of these anomalies were calculated incorrectly. Despite its lower atomic weight, Mendeleev immediately moved beryllium to the fourth position. Tellurium was categorized with oxygen.
In addition, Mendeleev did something else that his peers found utterly outrageous: he left gaps in the periodic table where, in his view, elements that have yet to be discovered should be included. In his paper, Mendeleev stated that “We must expect the discovery of many yet unknown elements, for example … aluminum and silicon, whose atomic weight would be between 65 and 75.”
The Basic Arrangement of the Elements
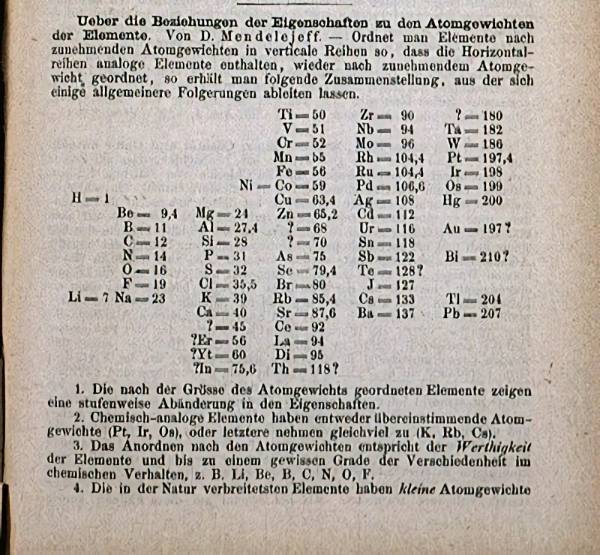
Mendeleev published his periodic table on March 6, 1869, just a few months before Meyer, under the title “On the Relationship of the Properties of the Elements to their Atomic Weights,” in which he also explained atomic weight changes and gaps and stated the laws that underlie his periodic table:
- When the elements are arranged according to their atomic weight, their properties exhibit periodicity.
- Elements that share similar chemical properties have atomic weights that are either nearly the same (platinum, iridium, osmium) or atomic weights that increase at regular intervals (eg, potassium, rubidium, cesium).
- The order of elements and groups in increasing atomic weight corresponds to their valences and, to some extent, to their properties.
- The atomic weights of the elements can be used to predict some of their distinctive properties.
For Mendeleev, it was obvious that his periodic system reflected the basic arrangement of the elements, effectively constituting the natural law of chemistry, rather than simply being an arbitrary order.
Mendeleev’s Elemental Predictions Caused Controversy
Mendeleev won the race when his Periodic Table was published. He was the first to be successful in both meaningfully arranging the elements and illuminating the patterns underlying their properties. But his contemporaries didn’t exactly applaud him for it. The exact opposite was the case.
“Unheard of and Unproven”
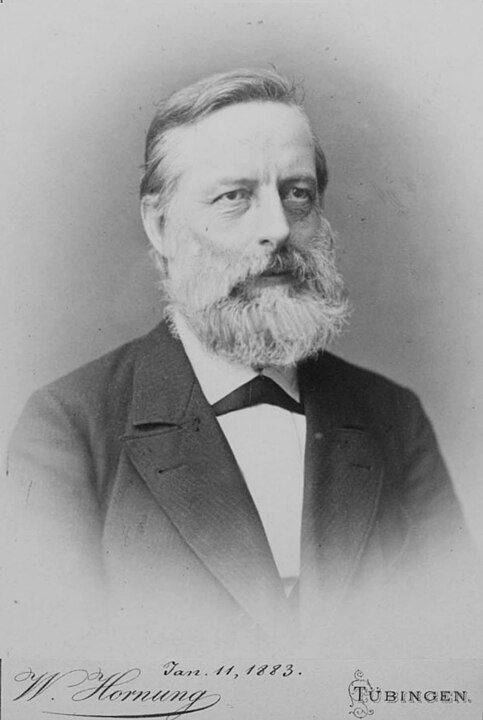
The responses ranged from apathetic coolness to outright rejection. After all, this Russian chemist from the far reaches of the country dared to virtually expose a number of his most illustrious contemporaries and predecessors, accusing them of producing inaccurate results. Such behavior was unacceptable. Meyer chastised his rival in front of the public for his “unjustified speculations.” Others predicted that his system would only last a short time because it would soon become outdated in any case. People were utterly unaware of what Mendeleev’s periodic table was about to bring to applied science.
Concerning the “Eka” Elements
Even so, Mendeleev refused to be deterred. According to Mendeleev, no law of nature, however fundamental it may be, was established all at once. Its laws were always preceded by numerous prejudices. Instead, in 1870, Mendeleev went a step further and made predictions about yet-to-be-discovered elements, saying that under each of silicon, boron, and aluminum, another element was still undiscovered and needed to be found.
Mendeleev named these elements Eka-aluminum, Eka-boron, and Eka-silicon after the Sanskrit word for “one” — “eka” — and had previously described their characteristics, including their atomic masses, specific weights, the types of salts they formed, and even the location of their melting points. Eka-aluminum, for instance, would be a silvery-white metal with an atomic weight of 68 and a density of 6. Again, Mendeleev received more derision for this than praise.
However, Gallium Does Exist
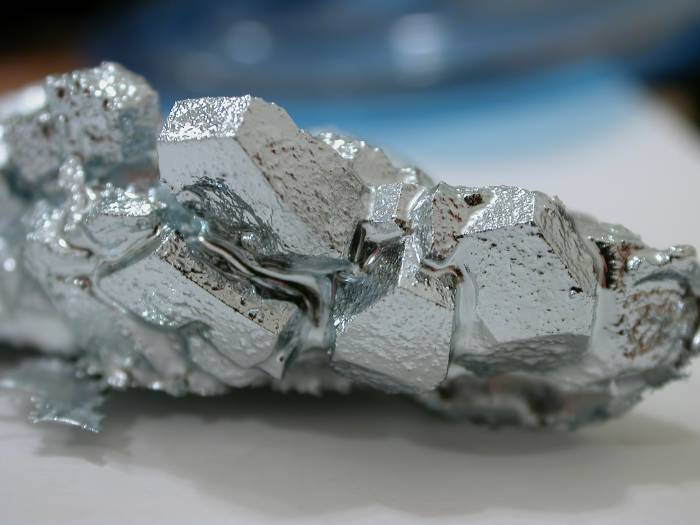
This would not alter until November 1875, five years later: French chemist Paul-Émile Lecoq de Boisbaudran found two violet spectral lines in a zinc ore’s emission spectrum that did not match any of the known element signatures. Soon after, Boisbaudran was successful in removing an unidentified silvery-white metal from the zinc blende. He gave it the name gallium, probably in tribute to his native France. He and others did not realize that his gallium corresponded precisely to the eka-aluminum predicted by Mendeleev until after he published his discovery.
Later, in 1879, the second element predicted by Mendeleev, eka-boron, was found by the Swedish chemist Lars Fredrik Nilson. German chemist Clemens Winkler made the discovery of the third element, germanium, in a mine close to Freiberg, Saxony, in 1885. Mendeleev had by this point, at the very latest, been not only vindicated but also formally acknowledged as one of the greats in chemistry.
But a few years later, something occurred that could potentially rock his entire periodic system once more.
Discovery of Noble Gases From Mendeleev’s System
Elements to Disrupt the Periodic Table
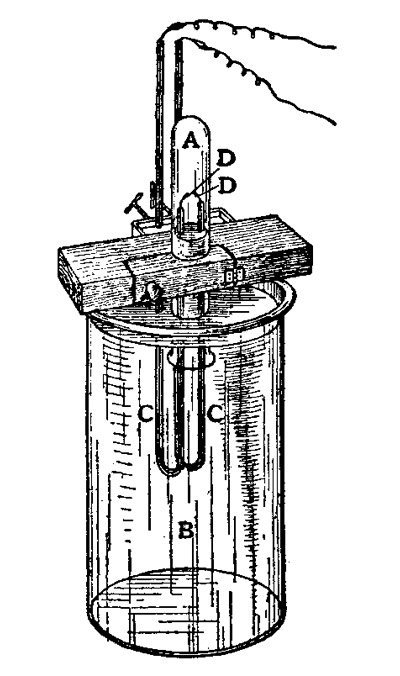
In a lab at the University of Cambridge in England in 1892, John William Strutt, Lord Rayleigh, a physicist, leaned intently over his workbench. In front of him, oxygen bubbled through ammonia liquid before vanishing into a copper tube that was extremely hot. The other end showed the emergence of much smaller bubbles. The gas that Rayleigh was worried about in this situation was nitrogen. When oxygen and ammonia’s hydrogen reacted, this is what was released.
In an effort to finally determine the atomic weight of nitrogen, Rayleigh had been attempting to measure the density of this gas more precisely than before for some time. Rayleigh repeated the test several times to make sure that there were no methodological flaws. He also employed a different measurement technique in which the nitrogen was taken straight out of the air by passing it over hot copper.
An Enigmatic Deviation
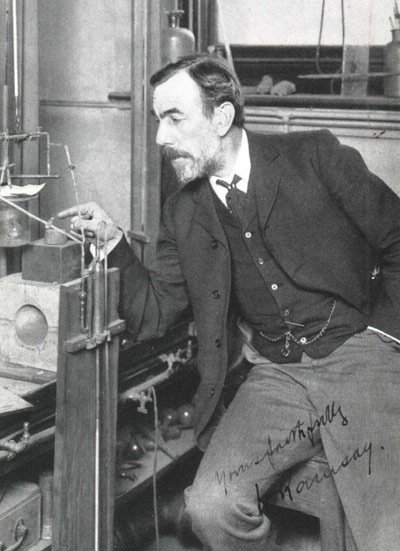
The physicist described how “again a series in good agreement with itself resulted” in his 1904 Nobel Prize lecture. According to him, the densities obtained by the two methods, had differed by a thousandth, which, though small, was completely beyond possible experimental errors. The nitrogen gas extracted from the ammonia was smaller in density than that from the air. And the question arose whether the difference could be attributed to recognized impurities.
Rayleigh consulted William Ramsay, a Scottish chemist conducting research at University College London, to address this issue. Both believed that the nitrogen in the atmosphere might contain an unidentified element. A tiny bubble of colorless gas, that was denser than nitrogen and could not be animated to react by anything, always remained, and this was confirmed by no fewer than two experimental approaches. But what was the point? Ramsay created a picture based on the spectral lines, or the chemical fingerprint, in spectroscopy, a relatively new technique.
Elements Without Space
The lines that emerged from the gas analysis were distinct from all known elemental signatures. It must be an entirely new, unexplored element. In honor of the Greek word “argos,” which means inert, Ramsay and Rayleigh gave the gas the name “argon” and made their discovery official on January 31, 1895. A closer look revealed that the atomic weight of argon was just under 40. Therefore, theoretically, it would be positioned in the periodic table between calcium (atomic weight 40) and potassium (atomic weight 39).
But of all places, Mendeleev did not discover a gap between alkali and alkaline earth metals. Was perhaps his periodic table incorrect after all? How could there be an element that did not fit into the scheme if he allegedly understood the law of elements?
Formation of a New Group
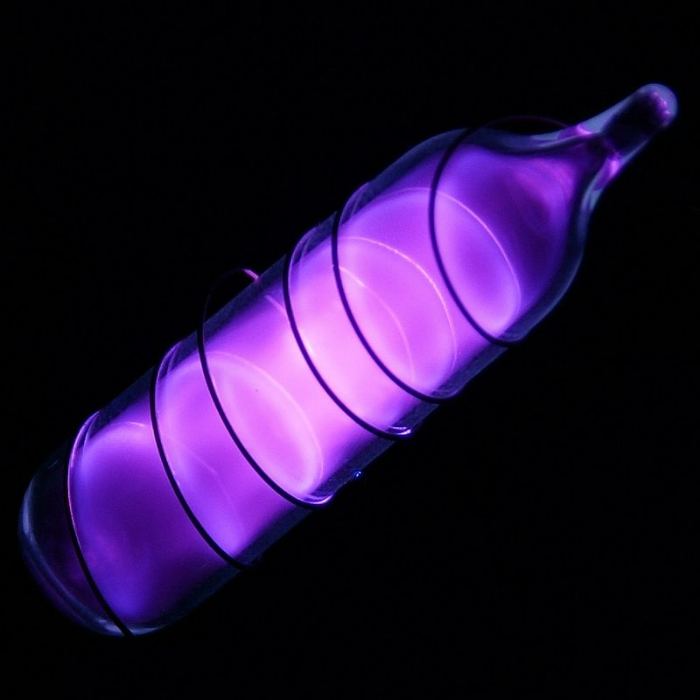
Nothing less than the overthrow of the recently established periodic order was threatened by an intense discovery. Ramsay, however, found a solution. As the gas didn’t want to interact with anything or anyone, he concluded that argon must have a valence of 0. As a result, it did not fit between potassium and calcium, which had different valences. It would instead be a member of a different, as of yet undiscovered group in the periodic table. Ramsay then positioned argon behind chlorine to form the eighth group of gases, the noble gases. However, there must be other elements in this group because no element in the periodic table could exist alone.
In fact, Mendeleev’s “Law of the Elements” turned out to be eerily prescient once more: Ramsay discovered in 1895 that a previously unidentified gas had been isolated from a uranium mineral in the USA and examined it in the spectroscope shortly after. The lines were made of helium, an element that had been seen in the Sun before but has not yet been classified. Ramsay had now established that helium was a noble gas as well because it was holding the group’s top position with an atomic weight of four. Ramsay found three more noble gases a few years later, naming them neon, krypton, and xenon, filling the eighth group.
The Periodic Table Today
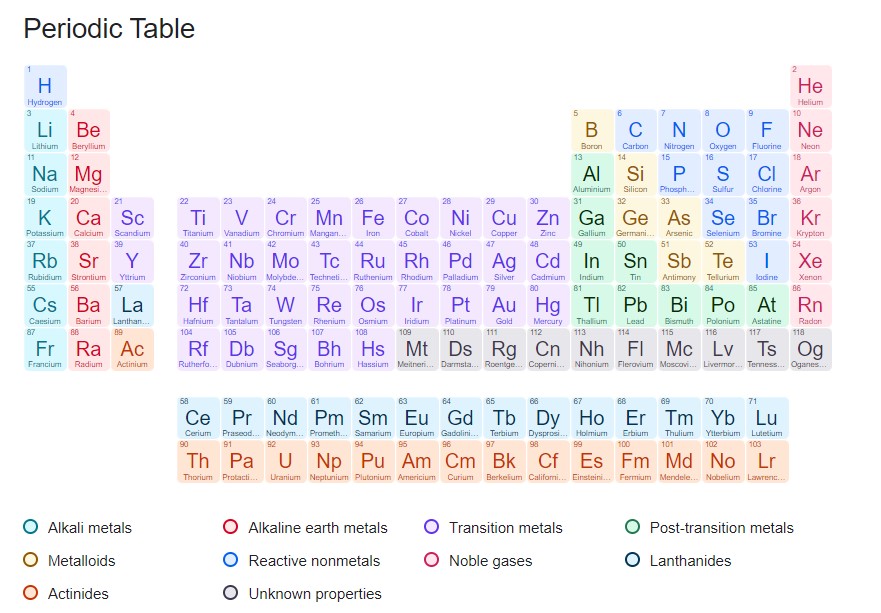
With the discovery of the noble gases, the Mendeleev-identified elemental order triumphed in its first trial by fire. The periodic table was now unshakeable, not even by the later discovery of yttrium and other rare earth elements. The laws that the Russian chemist discovered, however, are still in force today.
New Knowledge Reinforces the Old Order
Later discoveries have since upended entire worldviews in physics or biology, but Mendeleev’s “fundamental order of the elements,” the periodic table, has in fact turned out to be universal. Instead, a number of significant advancements in chemistry, from the structure of the atomic shell to the way that elements bond, have since supported his Periodic Table system.
Niels Bohr demonstrated in 1913 that the distribution of electrons in the atomic shell served as the basis for the bonding behavior of the elements by starting with the concept of valences, for instance. His discovery simultaneously gave physicochemical proof of Mendeleev’s theory that each group of elements in the periodic table has a specific number of electrons in their outer shells. They were largely responsible for the elemental properties, which in turn allowed Mendeleev to accurately predict the particular properties of as-yet-undiscovered elements.
Guiding Star for the Search for Elements
The “prediction” principle developed by Mendeleev is still employed by his successors today. Mendeleev’s periodic law is the guiding star. When the first artificial element was created in the 1940s, the big question was how to identify it and describe its properties. Because the International Union of Pure and Applied Chemistry (IUPAC) ruled that a newly discovered or created element was not officially recognized and named until its properties could also be described. For the extremely unstable and quickly decomposing transuranium elements, for instance, this is hardly feasible.
We now identify 118 elements, which means, there are 118 elements on the periodic table. It has proven to be quite easy to infer the properties of an element from its periodicity. Mendeleev and his contemporaries could not have imagined some of the exotic properties of those elements. And there are still more and more of them, produced in massive particle slingers under the harshest circumstances. Nobody is exactly sure where the upper limit is or how many more elements there could be in the periodic table.
Bibliography
- Döbereiner, J. W. (1829). “An Attempt to Group Elementary Substances according to Their Analogies”– Lemoyne College, New York, USA.
- Mendeleev, Dmitri (1869). “On the relations of properties of the elements to their atomic weights.”
- Boyle, Robert (1661). The Skeptical Chymist. London, England: J. Crooke. p. 16.
- Periodic Table of Elements – IUPAC | International Union of Pure and Applied Chemistry
- 1st periodic table is presented, March 6, 1869 – EDN