When a candle is lit, its shadow is cast on the window or wall in front of it. This shadow not only captures the outline of the wax body but also includes the image of the actual flame. It might seem like this is just the shadow of the light itself. You can better understand this phenomenon by observing a small object through the flame. This is because the flame’s transparency will vary in different spots.
An object positioned behind the central, brightly lit area of the candle flame seems nearly impossible to see. This part of the flame is the least transparent and plays a major role in creating the shaded edge on its surface. On the other hand, the outer edges and the portion of the flame surrounding the wick are highly transparent, resulting in minimal discoloration on the wall.
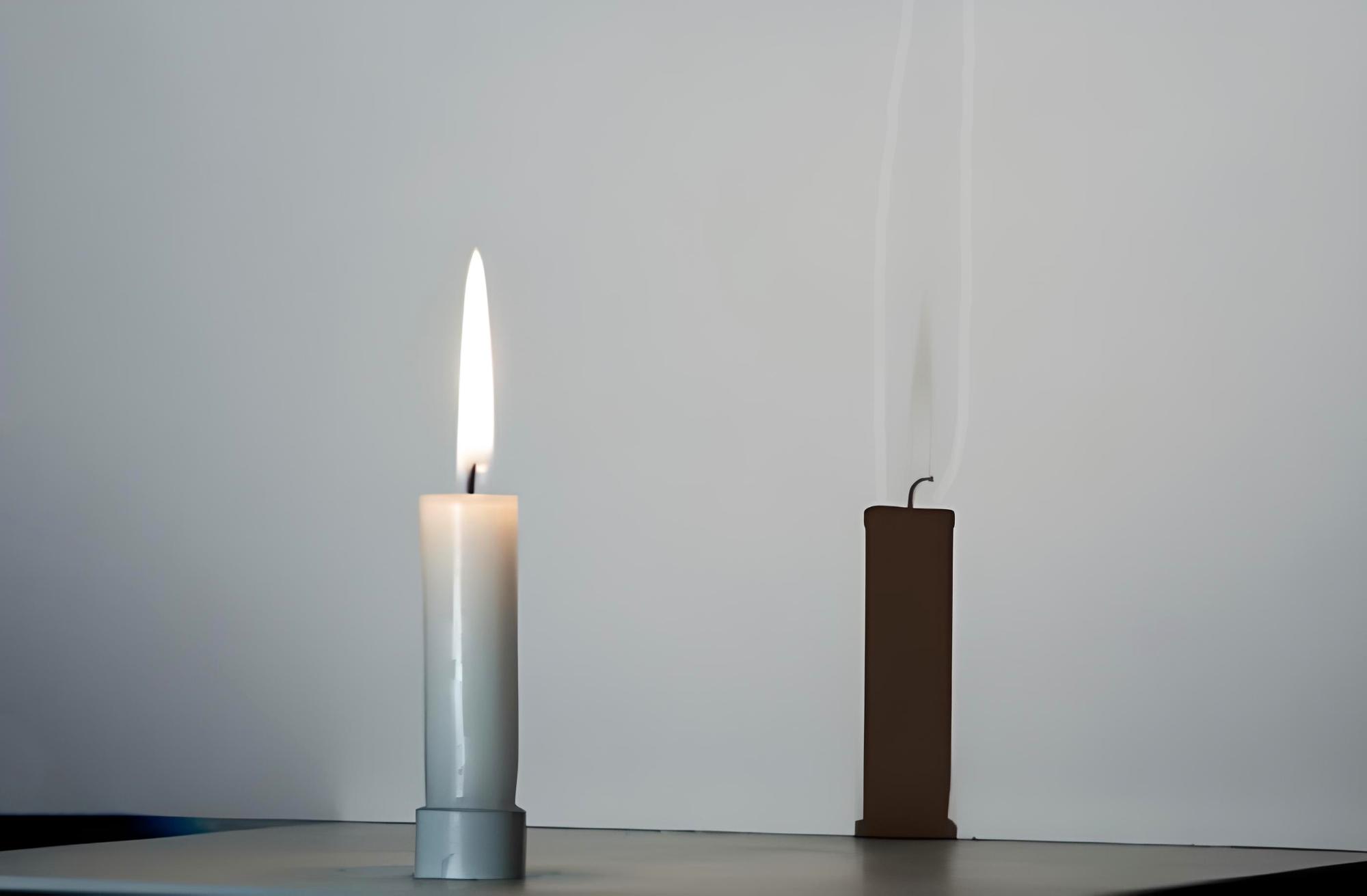
However, the projection offers more than just areas of darkness and light. It showcases bright patches that appear to shine even more intensely than the blazing sun. As a result, two parallel, brilliantly illuminated stripes flank each side of the flame, and a similarly radiant area stands out within the wick’s shadow. These heightened areas show that we’re not just seeing a candle’s shadow; rather, there’s a complex interaction between the flame’s light and the heated environment.
Numerous interconnected systems facilitate the intricate physical and chemical processes occurring within a burning candle. At the base of the wick, wax melts and transforms into long-chain hydrocarbon molecules as it rises. As these molecules move toward the center of the dark flame, they become hotter and break down into smaller fragments. They only ignite when they reach the outermost layer of the flame and come into contact with the oxygen in the air. This is where temperatures tend to rise significantly. The products of combustion are expelled in an upward plume due to the strong buoyancy effect.
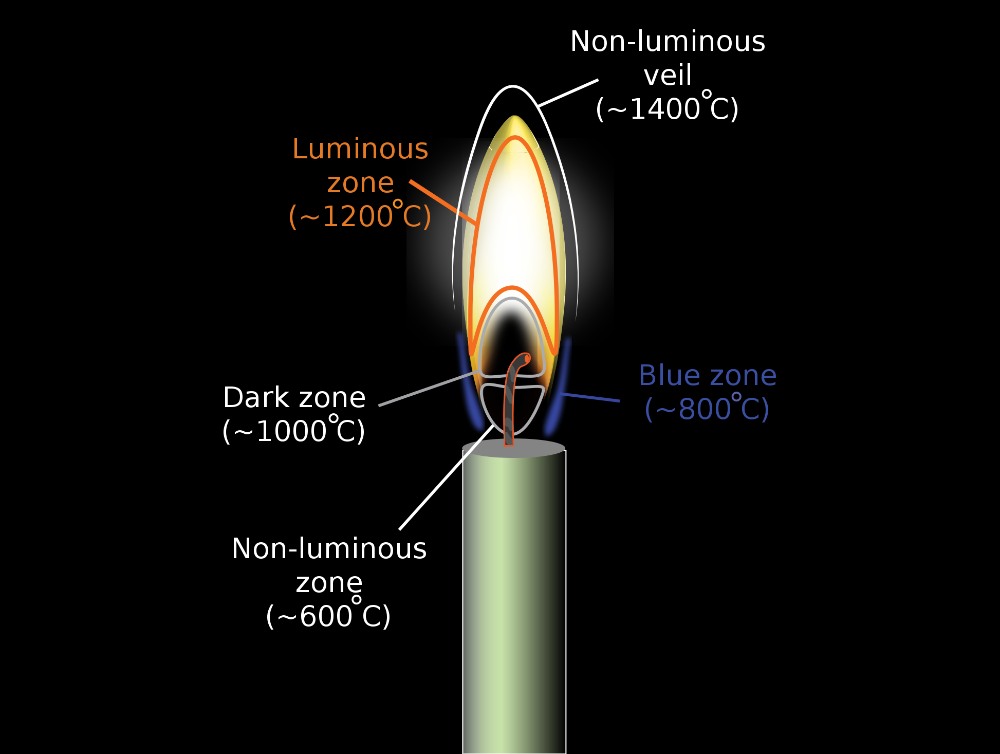
When outside air enters the exhaust gas plume, a significant change in temperature occurs. This change causes the bright lines we observe, and this phenomenon is a result of light penetrating the boundary layer. Changes in the gas’s temperature and density have an impact on this boundary layer, which causes refraction. Think of mirages on hot asphalt roads or flickering over a fire—both are examples of this effect.
The way light is refracted depends on the refractive index strength within the candle flame and the exhaust plume. Imagine tracing its path over the exhaust plume and the flame. Once, it travels on a plane right in the center of the luminous zone, and another time, it’s directly above the wick.
Now, this holds true for both planes: when approaching the flame from outside the exhaust plume, the refractive index drops extremely sharply within a radius of about 0.40–0.16 inches (10–4 mm) from the axis of symmetry. After that, the drop becomes less steep until it levels out. But from this point onward, the behavior of the two planes diverges.
As you move closer to the luminous zone, the refractive index starts to rise again, eventually leveling out in the center. However, things are quite different on the wick plane. Here, after hitting the minimum, the refractive index rises dramatically and even surpasses the value of the surrounding air.
This behavior can be expected due to the rapid temperature changes along the symmetry axis. Yet, the temperature profile doesn’t completely explain the high refractive index in the wick-to-flame-core area. The composition of the gas also has an impact on this phenomenon. The pure wax vapor present refracts light much more intensely compared to the air or combustion gases in the exhaust plume.
Gases having an optic impact
Light gets bent from its straight path in various ways due to changes in the refractive index. This happens at the meeting point of the surrounding air and the rising exhaust plume, impacting the area’s rays. The curved paths of these deflected rays eventually cross paths with the straight-traveling rays coming from a distance. As a result, the projection surface becomes adorned with striking bands of light.
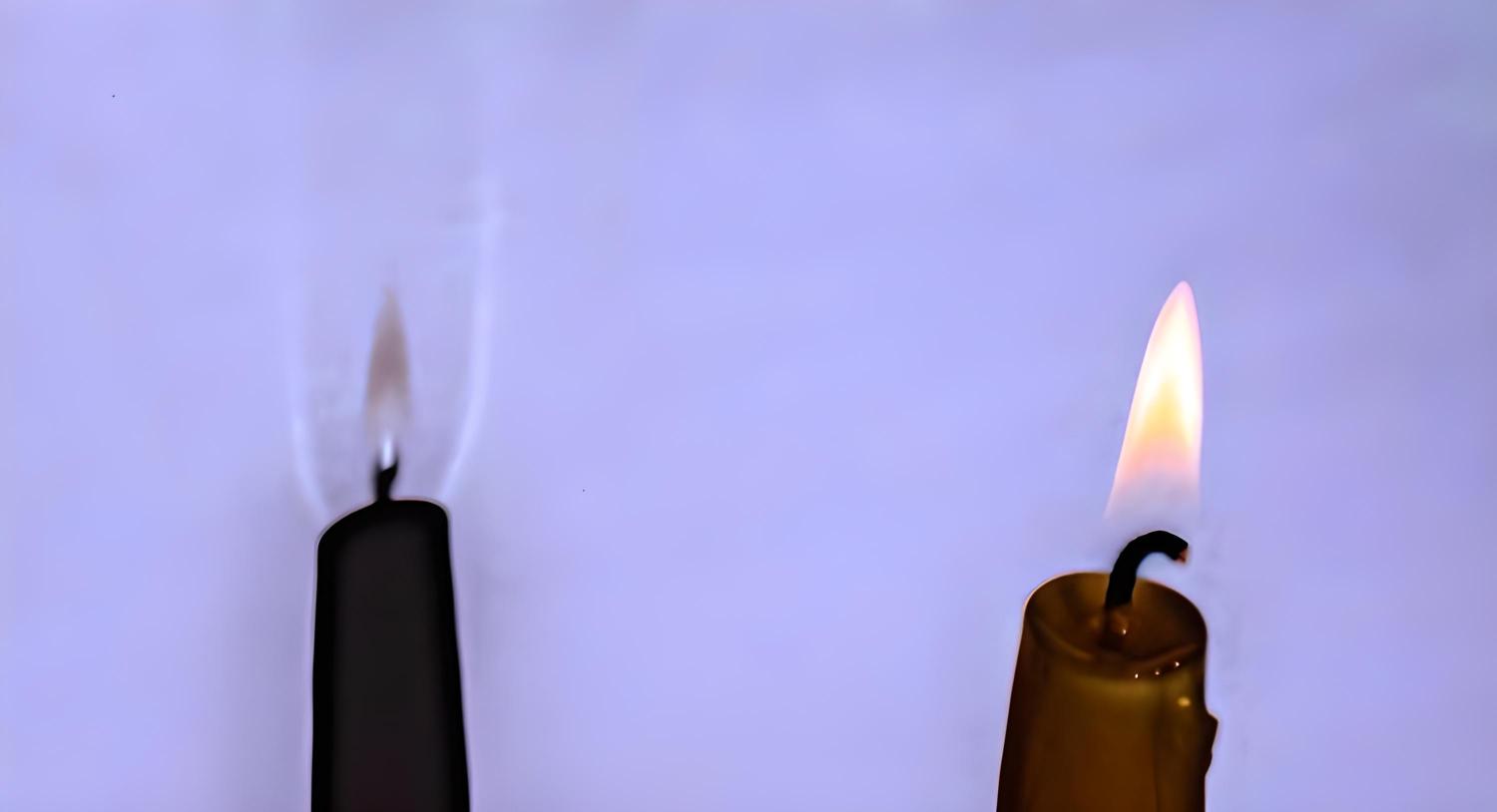
Furthermore, the rapidly increasing refractive index within the core of the flame causes light to be strongly deflected inward. To maintain symmetry, there’s some overlap to the right and left of the center. Consequently, the light intensity increases with distance in a specific region behind the candle. This explains how we’ve identified where the wick’s shadow is cast.
This indicates that the two stripes and central spot observed behind a backlit candle flame are connected to the refractive index profile. This is because of the high refractive index of the wax vapor near the wick and the existing temperature gradient.
For instance, the area with soot particles is where the candle flame is less visible. As a result, a shadow appears in the projection, although it’s notably brighter than the shadow from the candle’s central core. Interestingly, the shadow isn’t entirely gray; it holds a faint brownish tint.
The soot particles, which are about 20 nanometers in size and more than ten times smaller than the wavelengths of visible light, also have an impact on coloration. This leads to predominantly Rayleigh scattering, affecting the shorter-wavelength blue components of light. Consequently, the projection allows the significant long-wavelength reds to pass through. This creates a similar effect to early morning or late afternoon lighting.
References
- Can a fire have a shadow? – Wtamu.edu
- The Philosophy of Shadows – Oxford Academic (oup.com)