First, there was a whirling mass of gas and dust. And the Sun came into being first as a result of this, followed by the planets and their satellites. A new star was born in a vast cloud of gas and dust some 4.6 billion years ago. In little time at all, new planets began forming in its vicinity, ushering in the dawn of the solar system. Lucky for us, one of these newly forming planets had an orbit that placed it squarely inside the habitable zone, the same region in which our own Earth resides. But how did this happen, and how did our cosmic home transform into what it is now?
There is still a lot of mystery around the precise events that gave rise to our solar system and, by extension, our planet. Astronomers have to rely on their own understanding of physical principles and on hints gleaned from rock samples, the data collected by space missions, and the study of other solar systems. We can learn a lot from asteroids, too, as they are relics of the solar system’s infancy. All of these clues put us in a position where we can, at the very least, make some educated guesses about what happened, even if many questions still remain unanswered.
The formation of the Sun and its solar system
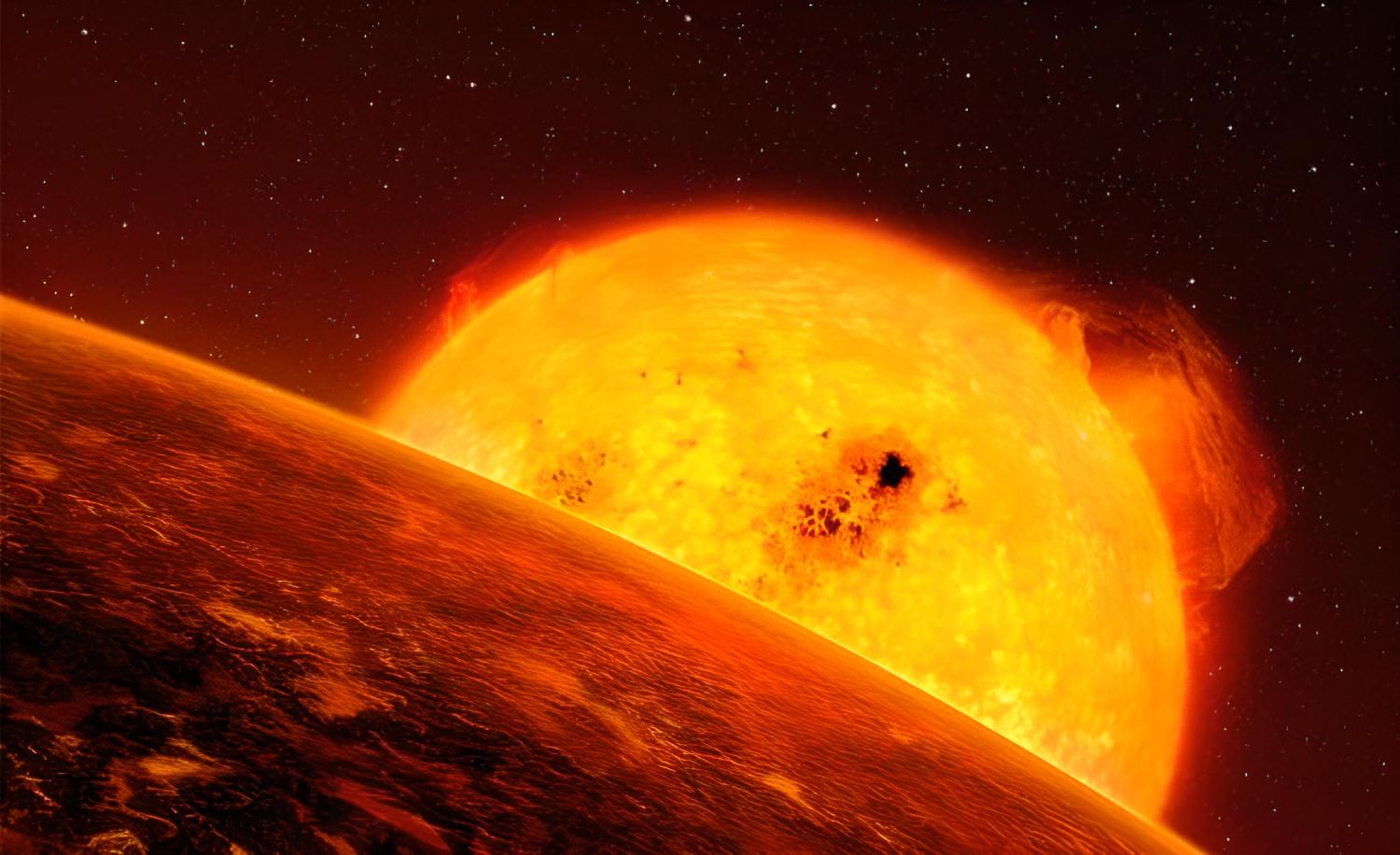
An early gas and dust cloud in Earth’s history. Hydrogen, helium, water vapor, carbon, and silicon compounds all made up a small fraction of the mass in this massive spinning disk. To some extent, the spinning of this so-called accretion disk resisted the effects of gravity and delayed or even prevented its collapse. However, the situation took a dramatic turn as a star in the vicinity exploded. Scientists calculate the timing of this supernova using measurements of oxygen isotopes in meteorites, which places it around 750,000 years before the birth of our solar system.
Trigger for nuclear fusion
The explosion caused shock waves that momentarily stopped the primordial cloud from rotating. The cloud eventually disintegrated because the centrifugal force was overwhelmed by the weight of the accumulating materials. The cloud’s core was where the majority of the gas and particles settled, becoming more concentrated there. The intense pressure caused the substance to become hotter. Extreme heat and pressure caused atomic nuclei to fuse together. A star, our young Sun, was formed when vast amounts of energy were released via nuclear fusion and radiated outward. Even now, its nuclear fusion continues to power the lights and heat of its environment. Inhibiting further collapse, the Sun’s rays maintain the cloud’s present state.
At 4.568 billion years ago, the still-revolving dust particles began to cluster together into bigger pieces, dubbed planetesimals. The gas cooled and condensed with time as well. As a result, silicon, iron, and nickel, among other volatile elements and compounds, accumulated in the inner area of the protoplanetary disk. The inner planets Mercury, Venus, Earth, and Mars progressively developed here by collisions with other pieces and accumulations of dust and smaller particles. But their surface was still hot and glowing, rather than solid. Ice, along with dust and gas, formed protoplanets in the outer region of the disk because heavier components are scarce in this region. Jupiter, Saturn, Uranus, and Neptune all descended from these massive planets.
The odd discrepancies in isotope
Though elemental makeups vary from planet to planet, all planets, including the Sun, are thought to have formed from a single primordial cloud. Therefore, in theory, they should all have the same ratios of atomic species, the isotopes, common to the solar system. Nonetheless, two separate studies found that this is not the case.
To do this, they analyzed samples of solar wind that NASA’s Genesis spacecraft had gathered over the course of almost three years and returned to Earth in a capsule. Because the outer solar envelope is thought to have retained essentially the same composition since its origin in the primordial nebula, the material that makes up the solar wind is regarded as an important remnant from the past.
When compared to the nebula the solar system emerged from, the composition of all terrestrial planets, meteorites, and comets in the inner solar system is atypical. There is less of the oxygen isotope O-16 on Earth, the Moon, Martian meteorites, and other asteroid pieces than there is in the Sun. Nitrogen isotope N-14 also varies between the inner planets, the Sun, and Jupiter’s atmosphere.
The ratio of oxygen to nitrogen isotopes in the area of the later inner planets was apparently changed by activities in the primordial nebula in the early days of the solar system. This may imply that the Sun and Earth were not generated from the same primordial nebula material.
From the protoplanets to the fully formed planets
Protoplanets first expanded their orbits around the Sun. Their gravity drew dust and debris into and along their orbits like giant vacuum cleaners. The surrounding protoplanets were also somewhat impacted by gravity, leading each to snuggle into its own orbit. The biggest planet in the embryonic solar system, proto-Jupiter, had a special influence over the other planets. The distance between it and Protomars was likely too small for another protoplanet to emerge. Instead, the area today is still home to the asteroid belt, a collection of both tiny and huge rocks.
A desolate, primordial cloud
The fact that Protojupiter follows an orbit around the Sun that gas giants like it typically avoid, is yet another way in which it deviates from the norm. Thus, today, the planetary clusters developed in specific regions while barren stretches of space appeared in others. Because according to a theory, the gas at a certain distance from a newborn star was evaporated by the Sun’s intense heat and light, leaving behind an empty space where no planets could form.
The Sun’s powerful gravitational attraction kept the closest stuff in place despite the high temperatures it generated. The hot gas dissipated into space farther away, where the force of gravity was lower. At that point, a gap opened up. However, at higher distances, not enough radiation arrived to damage the gas disk, so it again stayed unscathed.
Paradoxically, Jupiter’s orbit is in the exact spot in the solar system where the planetary gap is expected to exist. Exoplanet observations and simulations show that migrations like Jupiter’s are frequent in young planetary systems, thus, it’s plausible that the planet just migrated into its present orbit over time. In the future, astronomers hope to uncover answers to these and other puzzles regarding the early solar system via discoveries made in other solar systems.
Around a million years, after the planetary nebula cooled and planetary formation began, a powerful solar wind began to blow. The gas cloud’s remains were blown out of the system by the onslaught of radiation and charged particles. Inner, smaller protoplanets had insufficient gravity to keep their gas envelopes in place. At best, their initial atmospheres were quite thin, and they transformed into rocky planets similar to Earth. But the huge protoplanets toward the solar system’s periphery kept their gases for the most part. Today’s gas giant planets are the result of this event.
The beginnings of Earth
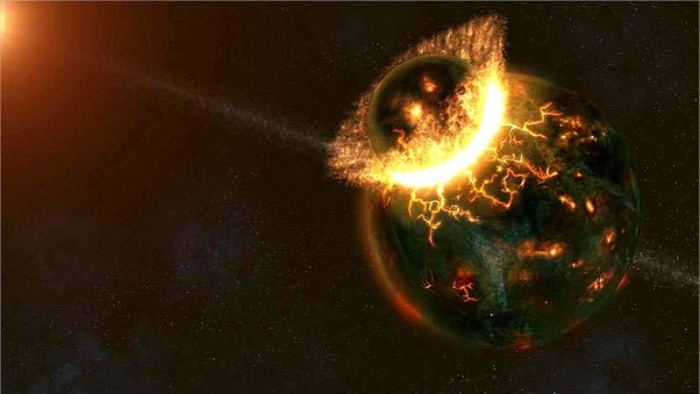
If we flashback 4.5 billion years, Earth was a seething mass of sticky lava, devoid of solid continents, seas, and a vital atmosphere—hardly an ideal place for life. In addition, it was still being attacked by both huge and tiny asteroids and planetoids from the space around it.
In the event of one of these impacts, an object about the size of Mars hit Earth, pulling off a huge piece of material, and this impact nearly destroyed the infant planet. However, Earth’s gravity kept the collision’s aftermath in orbit. The Earth’s Moon, a natural satellite, formed from them between a few hundred to a thousand years.
Perhaps another, smaller satellite also developed at this time, and fell to the Moon a million years later, causing the two to combine. Astronomers used computer simulation to verify this was theoretically conceivable. A possible explanation for the unusually thick crust on the lunar plateaus is that it is composed in part of the debris from a subsequent impact.
After all this turmoil, the inner solar system’s planet-forming leftovers were still being bombarded. Over and over, the persistent impacts injected vast quantities of energy into the developing Earth. In the meantime, the Earth’s mass was expanding slowly due to the falling debris. The greater the pressure within, the denser the core grew; therefore, the bigger it became, the more pressure it could withstand. This gradually raised the Earth’s temperature until its core reached above 3,600 degrees Fahrenheit (2,000°C).
Compositional differentiation of the magma ball
There was still a fair amount of internal homogeneity before this heating, and the chemical constituents were quite equally dispersed. However, when temperatures increased, the iron and silicate components that made up the Earth’s core began to melt. Because various metals had varying densities, this triggered a process of differentiation in which the heavier iron and a few other metals, nickel in particular, steadily sank into the Earth’s core. As time went on, they solidified toward the center of the Earth. Meanwhile, the Earth’s mantle and crust were composed of cooled molten rock made of silicate compounds that were carried to the surface.
Neither the precise composition of Earth before its differentiation nor the mechanisms involved in its formation are fully understood at this time. The issue here is that it is quite challenging to rebuild precisely how it formed into its current condition since we do not know what the mineral composition looked like at the beginning of the whole history. Significant progress was achieved in this direction in 2009 by geoscientists at the University of California, who used a computer model to recreate the distribution of iron isotopes in the Earth’s core prior to the formation of the Earth’s layers.
They did this by simulating changes in the ratio of iron isotopes in two minerals when the parameters of pressure, temperature, and electronic spin were varied. Following a month of computation, the model confirmed that the heavy isotopes, prompted by the high pressure, congregated towards the base of the crystallizing mantle.
From suffocation chamber to incubator
The Earth cooled down a little about 4.2 billion years ago. As a result of the Earth spinning faster than it does now, the average day length was just five hours, making life on the young planet tough. Now that the ozone layer had been depleted, the Sun was shining brightly and continuously, showering the surface of the Earth with potentially lethal ultraviolet radiation. Also, the big bombing wasn’t done yet: Meteorites continued to rain down on Earth, carrying with them carbon compounds and hydrogen, until roughly 3.5 billion years ago.
The valuable metals in meteorites
Even though these impacts were unpleasant for the young, uninhabited Earth, they could be responsible for a significant portion of the planet’s mineral riches today, including gold, platinum, and other precious metals. About 4.5 billion years ago, these metals should have been absorbed by the Earth’s core. But according to the scientists, the valuable metals only made it to Earth after differentiation, the process through which the heavy metals sank into the Earth’s center. Different isotopic patterns have been found in rocks formed before and during the Late Heavy Bombardment, which occurred roughly 4 billion years ago.
The Earth was fortunate enough to receive the majority of the valuable metals humans used and other vital industrial processes when it was struck by approximately 20 trillion tons of asteroid material. The deposits we see now are the result of the metals combining with rocks in the Earth’s crust throughout time.
The formation of the first atmosphere
Huge changes were occurring deep under the Earth, and it was fermenting and boiling below the surface as well. The so-called “first atmosphere” was created when gases and water vapor were ejected from volcanoes. The most recent scientific research indicates that it was mostly made up of the gases that the fire mountains are still bringing to the surface from deep under the Earth: water, carbon dioxide, nitrogen, and carbon monoxide.
As time went on, water vapor in the air condensed, and rain started to fall on Earth. This rain will last for the next 40,000 years. This first “deluge” gradually covered all lowlands in water, creating the first oceans. Carbon dioxide from the gas envelope dissolved in the nascent seas, accumulating into massive carbonate deposits. At the same time, this triggered still another shift in the atmosphere, one in which nitrogen replaced carbon dioxide as the primary gas and the greenhouse effect was weakened, leading to further cooling of the still-pretty warm Earth.
About 3.4 billion years ago, everything had finally come together, paving the way for the next crucial stage: The beginning of life on Earth. A new layer of nitrogen, carbon dioxide, and trace quantities of argon filled Earth’s atmosphere, joining the previously existing land and water. However, it offered little defense against the Sun’s harsh ultraviolet rays or the persistent meteorite strikes.
Despite Earth’s unfavorable conditions, the first signs of life were appearing on the planet. It’s still up for debate what precisely they looked like and where their components originated from—space or the ground.