Viruses are an excellent example of successful evolutionary adaptation since they outnumber all living cells, are present in all living things, and have been there since the beginning of life. But why are these parasitic cellular organisms so effective? And why do coronaviruses, Ebola, and influenza, all of which are enclosed RNA viruses, cause epidemics time and time again? It’s undeniable that viruses are quite effective in hijacking cells. They are biologically and structurally suited to infecting cells and turning them into replicating viral factories. Viruses have evolved an incredible range of tactics, each tailored to a certain host. However, it is plausible that a virus-like structure existed from the origin of all life, and that the cell parasitism of viruses was a key factor in the evolutionary breakthroughs that followed.
The first “virus”: Tobacco mosaic was originally attributed to what Beijerinck, in 1898, was the first to identify as a “virus“. He demonstrated that the inciting agent moved about in agar gel, making it clear that it was a ‘contagium vivum fluidum,’ or a soluble infectious agent, and not a ‘contagium fixum,’ like a bacterium.
Viruses are in every ecosystem, every living being
Viruses are the most widespread form of life on Earth, and they may be discovered in almost every environment. There may be ten to one hundred times as many viruses as there are live cells. However, just a tiny proportion of the viral variety has been discovered thus far.
Viral roommates
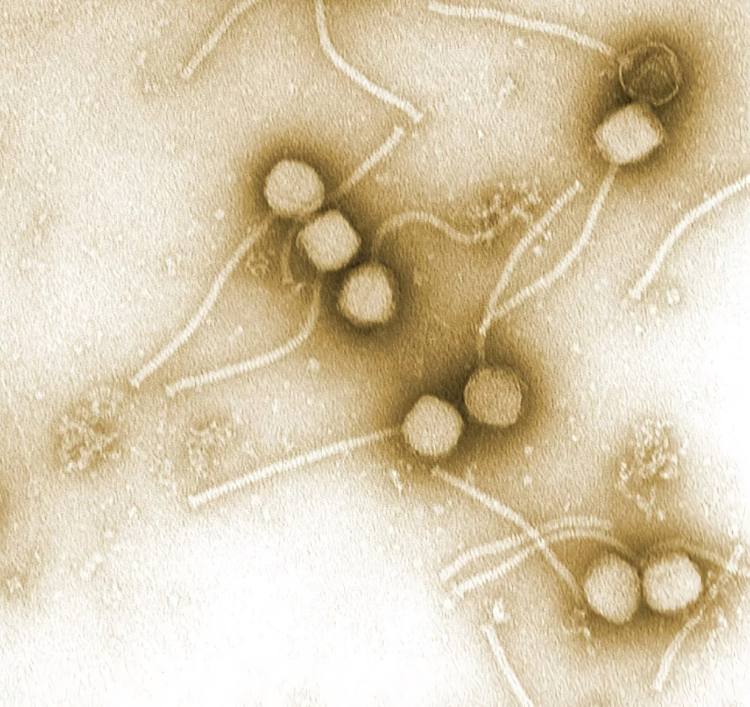
This includes habitats that are quite near to us, such as the air we breathe and the skin we have. After conducting a “dragnet search” for viral genes, scientists uncovered hundreds of millions of sequences. Around 90% of them have never been studied by scientists. However, the findings demonstrated that, like the bacterial microbiome, each region of the body has its own distinct population of viruses. And there are still viruses that haven’t been discovered that can infect our gut. Studies uncovered one of these pathogens, which affects the bacteria that live in the human intestine and goes by the name Escherichia coli.
Of course, we aren’t the hosts for the great majority of the viruses that live on and in our bodies; they are peaceful inhabitants. These viruses prefer to infect bacteria, which they then employ as a host for reproduction. Initial results suggest we may harbor millions more of these bacteriophages. The viruses that may really make us ill are a tiny fraction of this massive population. However, as the current Corona epidemic shows, the effects of these events on human health are far more severe.
Hosts throughout the family tree of life
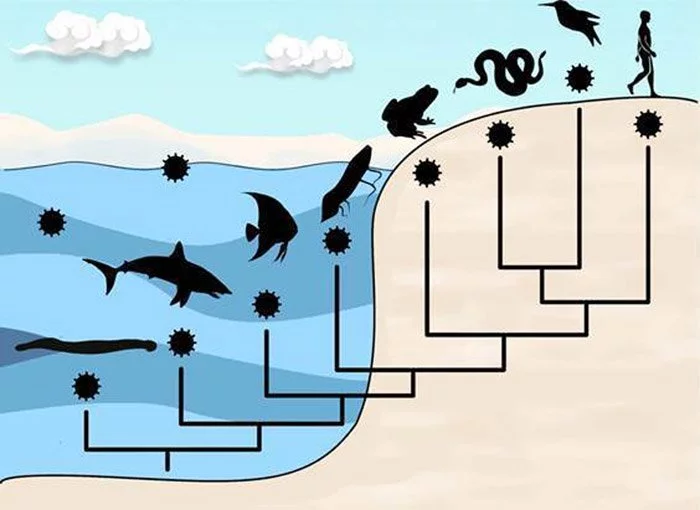
The host range of viruses, however, covers the whole tree of life, not just humans. Viruses may cause harm to almost every living thing. This diversity includes everything from nucleus-less archaea and bacteria to eukaryotic unicellular animals like insects and other invertebrates, all the way up to vertebrates like fish and humans. Aside from viruses that only infect algae, practically all higher plants have their own. At least in part, the tobacco mosaic virus, which causes illness in tobacco plants, was the first virus whose make up scientists were able to understand in 1935.
Nonetheless, most virus species are highly specialized, meaning they can infect just one host or a small group of closely related animals. Because they solely go after our resident bacteria, the millions of bacteriophages we harbor pose no threat to us. We are also immune to viruses that only attack plants and other invertebrates. Such barriers appear conceivable since creatures that are evolutionarily far away also vary in considerable detail in their cell biology. Since these conditions are so dissimilar, it’s challenging for a virus to reproduce in any of them.
From deep rock to the atmosphere
Viruses have been able to colonize practically every ecosystem on the planet due to the variety of hosts they may infect. They populate the watery environments of the world, from the tiniest puddle to our sewage to the grandeur of the seas, and the soils of practically every ecosystem on land. More than 10 million viruses may be found in just a single drop of saltwater. Viruses may be carried great distances in the air by both sea spray and desert dust.
As a consequence, more than 800 million viruses per square meter float in the atmospheric boundary layer every day. From a height of 2.5 to 3 kilometers, these airborne pathogens are carried by winds all across the world. It’s quite possible, the scientist continued, for a virus to spread through the air on one continent and then land on another. That’s also why related viruses may be discovered hundreds of kilometers away.
Viruses are ubiquitous, and may be found in even the harshest settings on Earth. Such places include Antarctica’s subglacial lakes, the hot springs and hydrothermal vents of the deep sea, and the rock strata at a depth of more than a kilometer below the ocean bottom. Based on sampling, in the deep biosphere, they may even constitute the majority of the biomass.
Viruses are ubiquitous, which demonstrates how extensive the virosphere, or viral universe, really is.
That begs the question, however, what exactly is their magic formula?
What are viruses?
Maximum efficiency in a minimal design
Viruses are, at their most fundamental level, just a bunch of DNA or RNA enclosed in a protein coat. In the natural world, they are the minimalists. Their basic structure is like a doghouse compared to the Sistine Chapel when a live cell is being considered. Nonetheless, this does not make viruses any less effective.
Highly variable genome
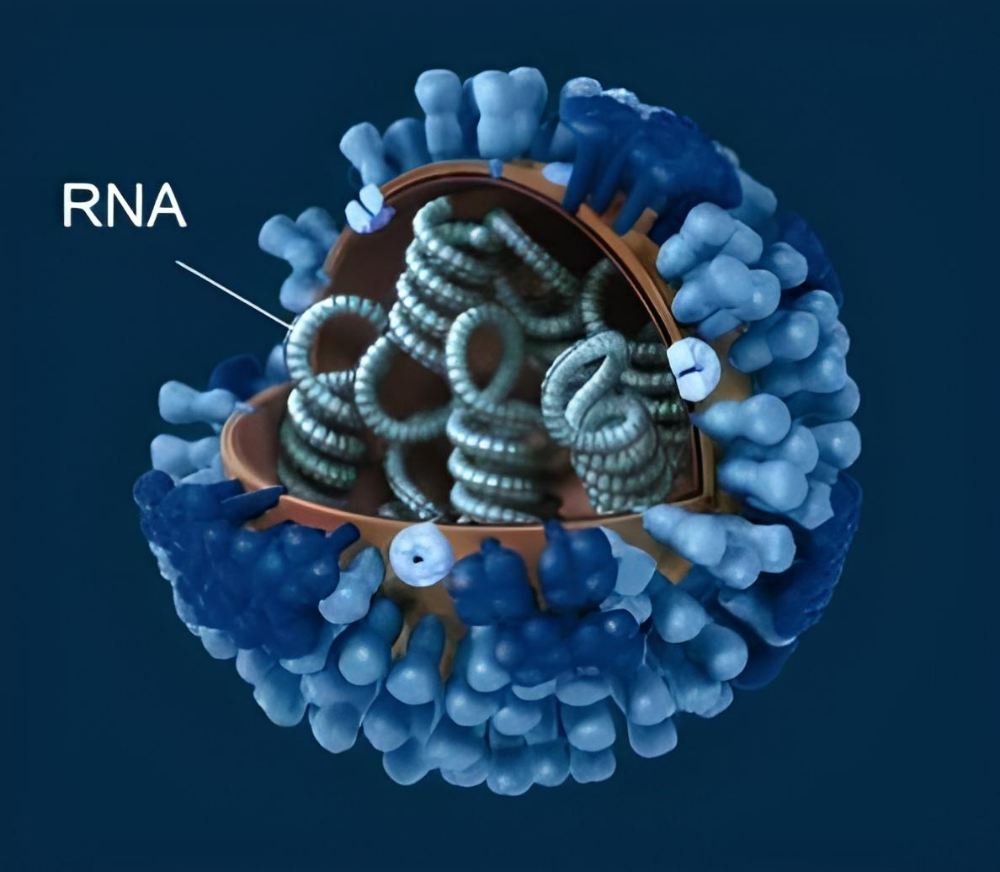
Viruses have evolved to fulfill their specific role in the world, and they carry with them all the tools necessary to maintain their species via reproduction. The viral genome encodes the proteins that make up the virus’s envelope and other critical enzymes. Genome size varies widely amongst different viral families, from tiny bacteriophages with just approximately 3,500 bases, to large polioviruses with 7,500 bases, to enormous viruses with 1.2 million bases.
Although all living things pass on their genetic information through the hereditary molecule DNA, the genetic material of viruses is very diverse. Depending on the kind of virus, it may be either DNA or RNA and can have either a single-stranded or double-stranded structure. Herpes viruses, papillomaviruses, and the smallpox virus are all examples of DNA viruses.
Many pathogens of serious and widespread infectious illnesses are RNA viruses, including the newly discovered coronavirus SARS-CoV-2, Ebola, measles, and influenza. Furthermore, many illnesses spread by mosquitoes or ticks, such as dengue, Zika, West Nile fever, and yellow fever, are caused by RNA viruses. The HIV virus, responsible for AIDS, likewise uses RNA as its genetic material.
Effectively built armored capsule
The viral capsule, or capsid, is the second part of every virus. It contains many copies of a single or small number of proteins and encloses the viral DNA. This results in a compact full capsid assembly that takes up little room in the viral DNA. However, viruses with just their capsid as an exterior shell are less adaptable because of their efficient manufacturing, which prevents the protein composition of the capsid from changing readily.
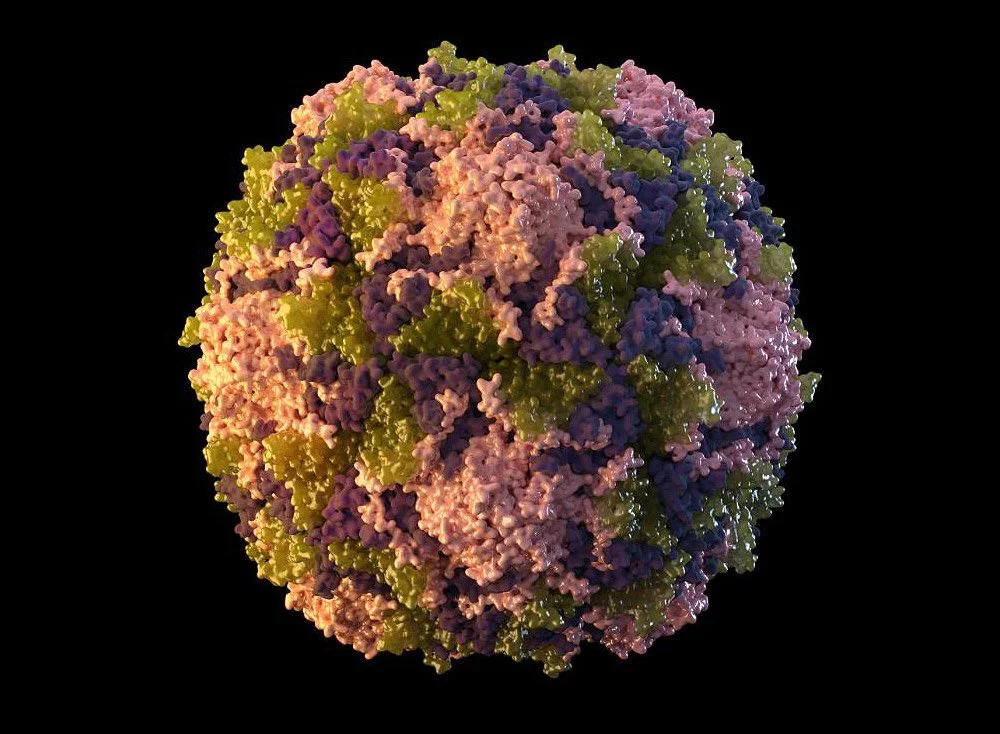
The capsid’s protein arrangement is another feat of natural efficiency: The proteins arrange themselves into a pattern of recurrent, identical basic units, which ultimately coalesce into the symmetrical viral capsid. Proteins may be thought of as disks that assemble into a helix, enclosing the viral DNA. The tobacco mosaic virus, the influenza virus, the measles virus, and the rabies virus are all examples of viruses possessing a helical capsid.
The icosahedron is another frequent capsid design; it has 20 sides and is built from basic units that are triangles composed of three copies of the same protein. The icosahedron has 20 of these triple units or multiples thereof, depending on the virus. Small and basic, its capsid is shared by the rhinoviruses and polioviruses that cause the majority of the colds in a country. They have a capsid made up of four distinct proteins, and each of those proteins creates 20 of these simple triple units.
Stolen envelope
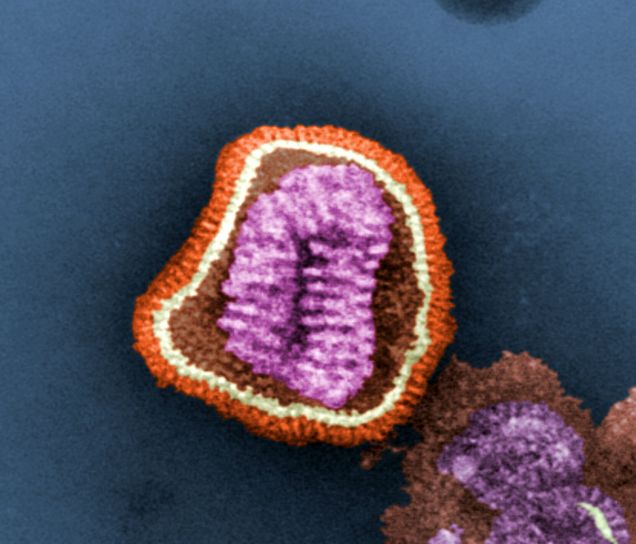
In addition to their DNA and capsid, many epidemic-causing viruses, such as the severe acute respiratory syndrome coronavirus 2 (SARS-CoV-2), also feature a viral envelope. It is similar to the cellular membrane in that it is a double lipid membrane with embedded proteins. The nucleocapsid is protected from the environment by this envelope, which completely encloses the virus.
Most enveloped viruses obtain the viral envelope by budding through the cell membrane and enclosing a portion of the host cell membrane around the virus, together with viral proteins already generated by the host cell. In other words, viruses may skip making their own blueprints for the double lipid membrane and instead just utilize the envelope. The membrane envelope facilitates the entry of these viruses because their envelope may readily fuse back to the cell membrane, releasing the mutant-filled capsid into the inside.
Why can enveloped viruses spread so easily?
Epidemics are often caused by enveloped viruses, such as the coronavirus SARS-CoV-2, influenza, smallpox, and Ebola. The viral envelope facilitates the ability of these viruses to alter their behavior in response to environmental changes, increasing their chances of infecting people.
Advanced camouflage
One reason is that many viruses have evolved a “camouflage coat,” in which the membrane of the virus’s coat and some of the coat proteins found inside it closely resemble those of common host molecules and cell components. This aids the pathogen’s efforts to hide from the immune defense system, increasing the likelihood that it will go undetected at first. Some coronaviruses, for instance, contain envelope proteins that are structurally similar to some human immunoglobulin G antibodies.
Many different viruses use sugar molecules produced inside the body as a disguise. Glycans, which are sugar molecules, bind to the proteins of the envelope and hide their terminal amino acids. Moreover, the virus’ sugar coats mimic those of the body’s own proteins, making it more difficult for the immune system to identify it as an invader. These glycans cover almost the entire surface of the HIV virus like a camouflage cloak; they account for over half of the total mass of the envelope protein.
Deception
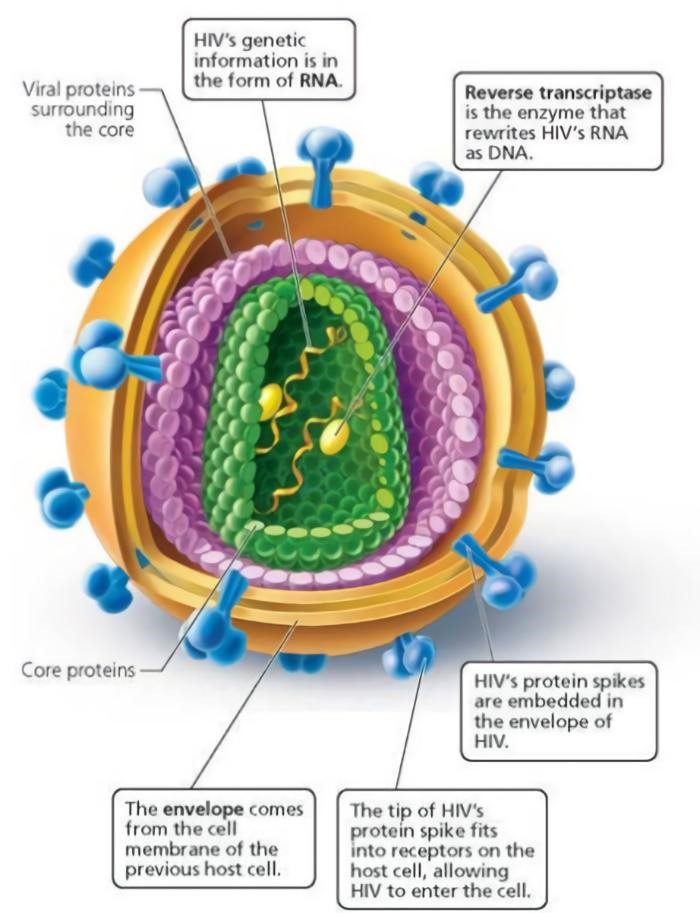
Additionally, viral coat proteins often exhibit protrusions or loop-shaped side chains near their termini. Although they are subject to alteration as a consequence of mutations in the virus’s genome, these components are not essential to the pathogen’s operation. As a result, it is able to rapidly and regularly alter its outer structure. This renders the immune defense antibodies useless since they no longer have any places to recognize or dock with the pathogen.
The Ebola virus utilizes a more ingenious strategy: it creates “decoys,” or little bits of a membrane with linked protein sections, to lure the host’s antibodies and divert them from the true viruses before they leave the host cell.
Precision docking
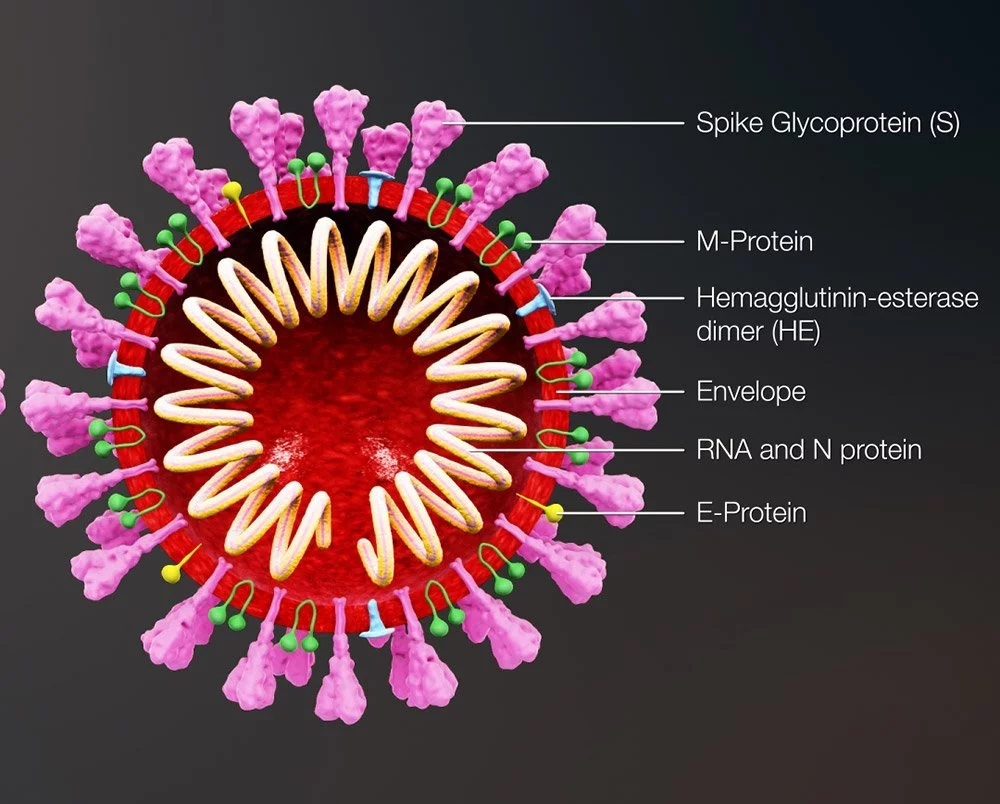
Enveloped viruses may rapidly adapt to new hosts and transcend the species barrier from animals to humans, which is part of the reason why they are so effective at causing new epidemics. Certain viruses, such as influenza and coronaviruses, employ components of an envelope protein to attach to particular receptors on their host cells, while non-enveloped viruses have a more difficult time modifying their capsid.
The virus can only infect cells if its binding site is properly configured. This also affects which organs or tissues a virus infects, since not all cells have the same receptors. For instance, the SARS-CoV-2 coronavirus’s spike protein is shaped in such a way that it docks with the ACE2 receptor on human respiratory and lung cells. In contrast, the TBE virus that is responsible for tick-borne meningitis has a binding site that is specific to the receptors found on nervous system cells.
Predestined for the origin of the species
In any case, the binding site of encapsulated viruses is very malleable and may undergo rapid morphological shifts due to mutations. Because of this, these diseases are able to modify their cell receptors to interact with those of new hosts. Additionally, they are able to communicate with people of various species. Most infectious illnesses that have recently migrated from animals to people are caused by enveloped viruses, and this is not a coincidence.
Recent examples include SARS-CoV-2, a coronavirus that developed in bats but then underwent a series of modifications that made it transmissible to humans. After infecting another species, perhaps pangolins, it made the leap to people in China in late 2019. There is debate about whether the coronavirus fully adapted to its animal hosts or to its initial human hosts.
Moreover, influenza viruses are proficient “species barrier jumpers.” Many of the influenza viruses now making the rounds first appeared in birds, and new avian influenza strains are constantly emerging, some of which have begun to show symptoms of adaption to humans. Furthermore, the 1918 influenza pandemic virus also spread from birds to people. In 2009, its offspring were responsible for another pandemic, this time caused by the “swine flu.”
How do viruses multiply?
Viruses can only replicate inside living cells. They can only reproduce by taking over the cells of other organisms and using their resources for their own ends. They lack the ability to generate their own genome or envelope and lack the metabolic machinery to manufacture energy-rich molecules like ATP. They must always live within a host cell.
DNA viruses: taking over the cell machinery
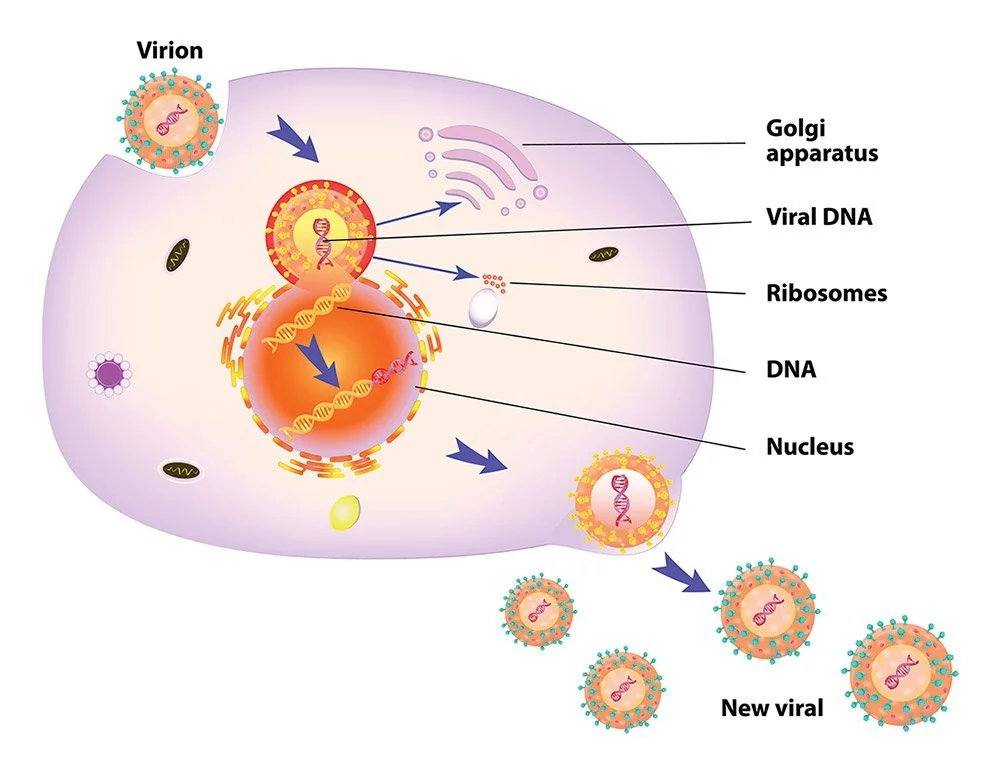
When a virus invades a cell, it immediately starts its aggressive takeover. Infectious agents like herpesviruses, papillomaviruses, and others that employ double-stranded DNA to replicate do so by transporting their DNA into the nucleus of a host cell. They trigger the cell’s own enzymes to decode the viral DNA and produce mRNAs. After leaving the nucleus, these mRNAs make their way to the ribosomes, where they are used to make viral enzymes.
The second phase involves the virus using these enzymes to totally take over the cell and turn it into a viral factory. At the same time as the proteins for the capsid and the viral envelope are being made on the ribosomes in the nucleus, fresh copies of the viral genetic material are being created in rapid succession. During budding, the viral envelope encloses the newly formed virus after it has penetrated the cell membrane and linked the viral genome to the capsids. The aftermath is a dead cell and a flood of new viruses.
Coronaviruses: multiplication with its own RNA factory
The coronavirus has a unique method of reproduction. This is due to the fact that instead of DNA, it has a single strand of positive RNA, which has both benefits and drawbacks when it comes to gaining control of the cell’s machinery. The downside is that, since our genome is stored as DNA, our cellular machinery was not developed to duplicate RNA. The only purpose of RNA is to transport genetic information from the nucleus to the ribosomes. The ability to replicate RNA into RNA is not supported. In order to replicate, the coronavirus must first set up its own duplicating machinery within the host cell.
However, once inside the cell, a portion of the virus’s RNA strand may make its way to the ribosomes, where it can be read without any additional processing. From this, the primary protease is created and used to break down the lengthy protein chain generated by the ribosomes.
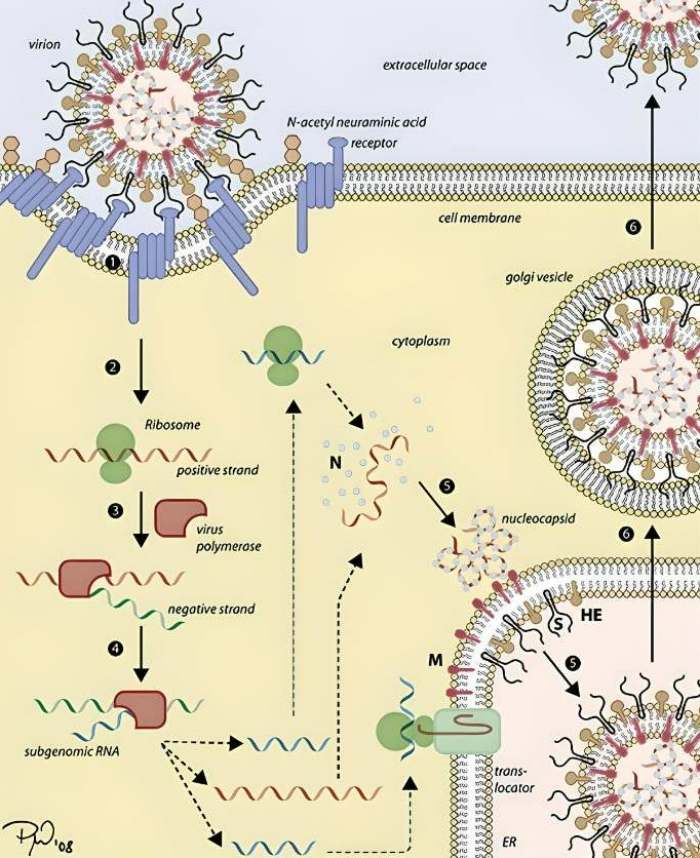
This generates the mechanisms necessary for replication, turning the cell into a production facility for more coronaviruses. The process begins with the viral enzyme known as polymerase copying the virus’s original RNA strand in order to create a complementary strand. This strand of negative RNA is currently being used as a transcription template to generate an abundance of new, positive RNA strands, which will ultimately act as the viral genomes. At the same time, the ribosomes read a short section of the opposite RNA strand and synthesize the capsid and new viral envelope proteins.
Copying errors make RNA viruses more adaptable
In addition to coronaviruses, this replication method is used by all other positive single-stranded RNA viruses. Dengue, yellow fever, chikungunya, Zika, and West Nile viruses are only some of the examples; so are rhinoviruses, rubella, the poliovirus, and the virus responsible for foot-and-mouth disease in animals. It is generally agreed that RNA viruses are the most probable causative agents of new zoonoses and pandemics.
This is not by chance; RNA viruses lack these error-checking and -correcting processes because they are unable to employ the cell’s regular replicating machinery. Therefore, many more mistakes are made while making new viral RNA than when making DNA viruses or replicating cells.
However, this also implies that RNA viruses are able to undergo mutations and develop at a far quicker rate than other pathogens. This is a key feature that helps viruses adapt to tough situations, such as the body’s immune system or antiviral medications. RNA viruses may also rapidly adjust to different hosts and docking sites in cells. As is the case with coronavirus, mutations often alter the spike protein and, by extension, the binding location to host cells. That’s why coronaviruses are so excellent at crossing from one species to another.
How viruses may have moved life forward
During pandemics, viruses especially don’t have a favorable rep. We often see them in our minds as dangerous, invisible foes that spread illness. However, there is another side to our viral housemates: they may have been responsible for driving the development of life or possibly making it conceivable.
Around the size of a bacterium
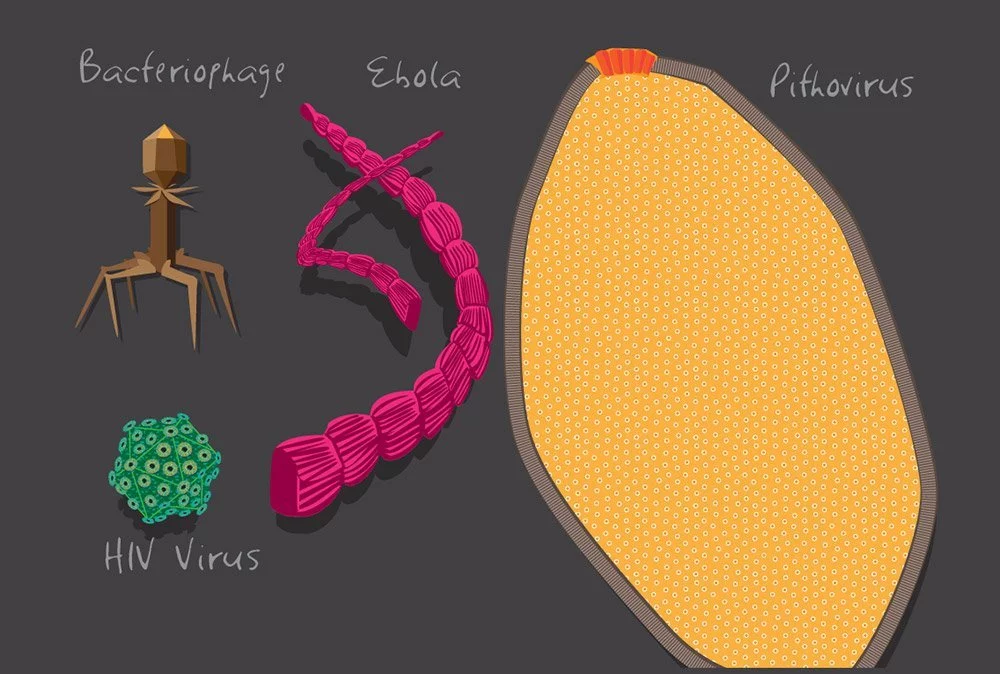
The discovery of enormous viruses, which are just slightly larger than some bacteria, provides a possible explanation. Some amoeba-associated viruses, such as pandoraviruses and megaviruses, may grow to a diameter of 700 nm, making them about twice as large as chlamydia-related bacteria. Not only that, but their genome is just as large as that of the Klosneuvirus, which was found in 2017 at an Austrian sewage treatment facility. The genome of the norovirus (found around toilets), which causes genital herpes, is 1.54 million bases long, or almost three times as long as that of the bacterial pathogen Mycoplasma genitalium.
What is contained in the DNA of these gigantic viruses, however, is much more peculiar. This is because viral DNA includes genes for protein biosynthesis, the process by which cells manufacture the components for their metabolism and reproduction, in addition to the instructions for the construction of new viruses. It follows that these viruses at least have the genetic instructions for a lot of this cellular apparatus.
Viruses in the primordial soup
How, therefore, do these genes get into the body? To be more precise, both of the following scenarios are theoretically possible: First, it’s possible that these and other viruses originated from regressive single-celled creatures. Because of this, it is likely that viruses and all creatures with cells have a common ancestor. Then, when the viral forerunners transitioned into obligatory cell parasites, they reversed their development to eliminate all the parts of the cell they no longer required.
Alternatively, viruses’ basic architecture might be traced back to the origins of life. Many scientists have speculated for a long time that RNA, not DNA, served as the hereditary material in the earliest cell-like structures that formed in the “primordial soup.” This suggests that modern RNA viruses may have descended from ancient RNA viruses.
Suppliers of new genes
Nonetheless, viruses may have played a pivotal role in the latter stages of evolution. The reason for this is that many of them can integrate their DNA into the host cell’s DNA. Occasionally, important sequences from these viruses are passed on to the next generation. Protein structures of viral origin have recently been detected in practically all domains of life, from bacteria to eukaryotic plants and animals.
Our genome also contains viral gene sequences; in 2001, when the first human genome was decoded, researchers were shocked to discover 600,000 base sequences in our genome that were definitely of viral origin. Approximately 43% of our overall genetic material is now known to be composed of such viral DNA sequences, and some of these sequences have been shown to serve essential roles. Placental development, hormone generation in the adrenal glands and ovaries, protection against cancer, and proper muscle and brain function are just a few examples of how viral genes play an important role in the body.
We should thus see viruses as a source of novel genes for cellular organisms, and not only as pathogens. Scientists think that viruses have helped cellular organisms evolve by transferring genes to them at various points throughout time. Simultaneously, some of these viral sequences may possibly be older than the period when viruses and cells had a common ancestor.
Facilitator of evolutionary change
Indirectly, viruses also pushed evolution forward by selecting novel features. Ten thousand mammalian proteins were compared for their rates of evolution in 2016. Therefore, it was clear that the rate of change for proteins involved in virus defense or other kinds of viral interaction has been three times that of most other proteins.
Viruses seem to be responsible for around 30% of all amino acid modifications in the primordial section of the human proteome. This is the first proof that viruses play such a significant role in shaping adaptability. In spite of this, researchers find that encounters with viral infections mold not just our immune responses but also millions of additional proteins with seemingly no link to viruses or the immune system.
Viruses pose a serious danger to human health, yet without them, the quality of life on Earth would likely suffer.
Bibliography
- Nationalgeographic.com, 2020, “There are more viruses than stars in the universe. Why do only some infect us?“
- “Virus Taxonomy: 2021 Release”. talk.ictvonline.org. International Committee on Taxonomy of Viruses.
- Breitbart M, Rohwer F (June 2005). “Here a virus, there a virus, everywhere the same virus?”. Trends in Microbiology. 13 (6): 278–84. doi:10.1016/j.tim.2005.04.003.
- Koonin EV, Senkevich TG, Dolja VV, 2006. “The ancient Virus World and evolution of cells”. Biology Direct. 1 (1): 29. doi:10.1186/1745-6150-1-29.
- Lawrence CM, Menon S, Eilers BJ, Bothner B, Khayat R, Douglas T, Young MJ (May 2009). “Structural and functional studies of archaeal viruses”. The Journal of Biological Chemistry. 284 (19): 12599–603