White Dwarfs: When Smaller Stars Turn Into Corpses
When normal stars like the Sun run out of fuel, they collapse into white dwarfs. These dense stellar corpses are the last stage of development for low and medium mass stars.
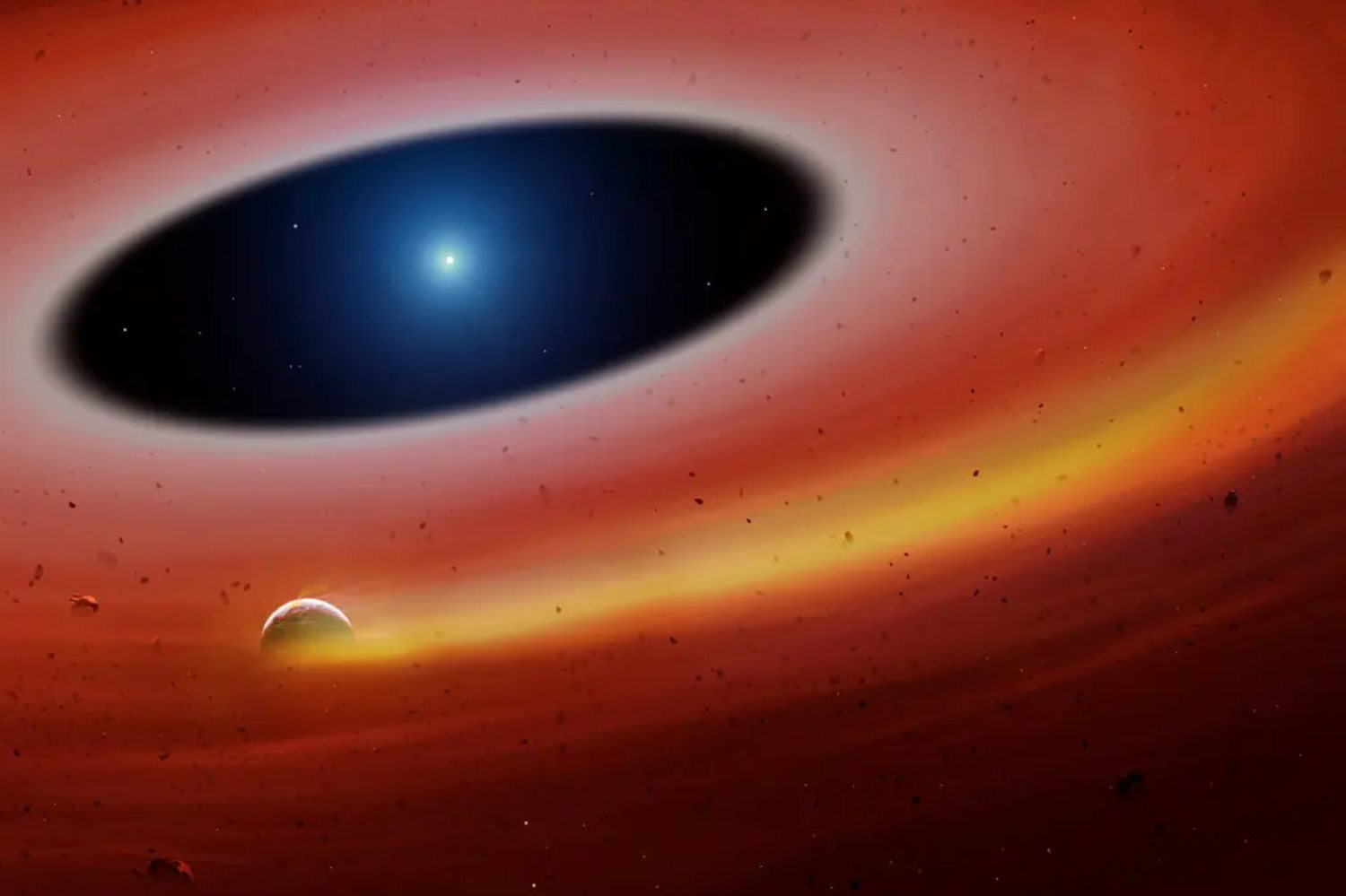
When normal stars like the Sun run out of fuel, they collapse into white dwarfs. These dense stellar corpses are the last stage of development for low and medium mass stars.