Without catalysts, our world would look very different: Many chemicals, pharmaceuticals, and everyday products would not exist without these reaction accelerators. But the optimal catalyst has not been found everywhere. Catalyst research is therefore one of the “hot” topics in chemistry today.
Without catalysts, not only would car exhaust gases be dirtier, but many things around us, perhaps even most of them, would not exist at all: no plastics, fewer medicines, and no artificial fertilizers. Catalysts also help to reduce emissions that are harmful to the climate. So we owe a lot to reaction accelerators.
It is estimated that catalysts are used in 80 to 90 percent of all chemical processes. The products created with them contribute up to one-third of the world’s gross domestic product. They are one of the most important technologies in human history.
What are catalysts good for?
The history of catalysis is practically as old as that of the chemical industry. Even without a theoretical background and without knowing the mechanisms involved, practitioners learned early on that many important reactions take place more quickly under certain conditions.
One of the earliest examples of industrial catalysis is the lead chamber process developed in 1746 for sulfuric acid production. In this process, nitrogen dioxide catalyzes the oxidation of sulfur dioxide to sulfur trioxide. In the contact process developed in the 19th century, first platinum and later vanadium pentoxide acted as catalysts.
The facilitators
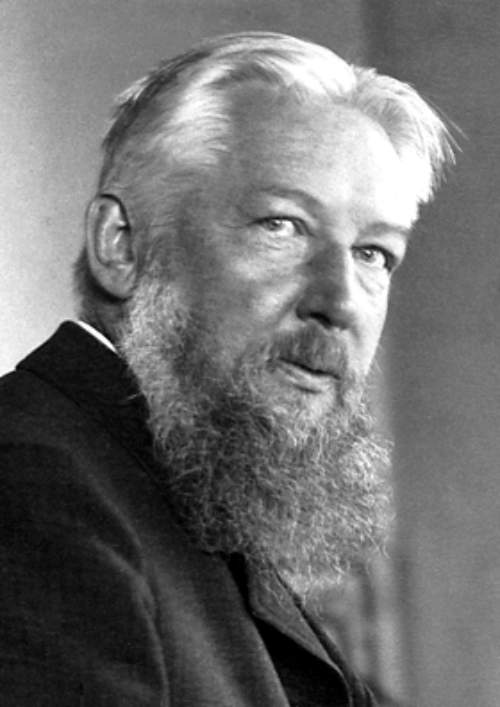
Whether it’s chlorine, nitric acid, plastics, methanol, the blue dye indigo, or ammonia, which is important for fertilizer production, catalysts make it possible to achieve significant conversion rates in the synthesis of countless important chemicals. One of the earliest definitions of catalysis came from Wilhelm Ostwald, who, at the end of the 19th century, had intensively studied the influence of catalysts on chemical reactions. In this, he already took the effect on the reaction rate into account.
Later, he derived from this the description that is still valid today, according to which a catalyst is a substance that increases the speed of a chemical reaction or makes it possible for a reaction to take place without itself being consumed. Ostwald compared this effect to the effect of lubricating oil on the wheels of a clockwork. In both cases, existing resistances would be reduced: in the clockwork, due to mechanical friction; in the chemical reactor, due to the energy barrier. Ostwald was awarded the Nobel Prize in Chemistry in 1909 for his fundamental work.
What can a catalyst do—and what cannot it do?
Important in this context: A catalyst can only accelerate or enable reactions that are also thermodynamically favored, i.e., in which the energy level of the products is lower than that of the reactants. Equally important, the thermodynamic equilibrium of a reaction is not influenced by catalysts. A characteristic feature of a catalyst is that it reduces the activation energy that must be expended to break existing bonds so that a new state with new bonds can be created.
Typically, catalysts lower this energy expenditure by enabling particular transition states that can be achieved with less energy input. They do this, for example, by temporarily forming covalent or hydrogen bonds with one of the reactants and thus influencing its reactivity.
Incidentally, “not being consumed” is not always true in practice. Particularly in heterogeneous catalysis, in which the catalysts are usually applied to a solid phase, changes do occur in the course of the process, such as coking or sintering. Operators of such processes must therefore renew their catalyst material from time to time.
What is asymmetric organocatalysis?
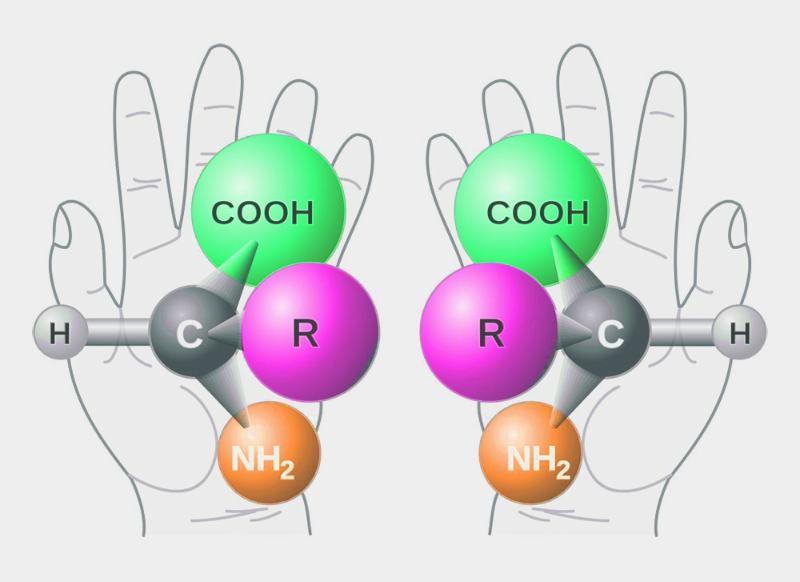
A look at Stockholm proves how significant catalysis is for chemistry. Since 1909, a total of ten Nobel Prizes in Chemistry have been awarded for catalysis research in the narrower sense. Among the laureates were such well-known chemists as Fritz Haber and Carl Bosch. Six of the ten Nobel Prizes for catalysis have only been awarded since 2000, demonstrating how “hot” this field of research still is.
The last in the illustrious line of Nobel Prize winners in 2021 were Benjamin List of the Max Planck Institute for Coal Research in Mülheim and David MacMillan of the University of California at Berkeley. They received the prestigious prize “for the development of asymmetric organocatalysis.” Around the turn of the millennium, both researchers, independently of each other, opened the door to this new specialty field of catalysis.
Chemists speak of “organocatalysis” when the catalysts are organic molecules. It has long been known that these can accelerate chemical reactions. Justus von Liebig already made use of this almost 200 years ago when, among other things, he used cyanide as a catalyst for the formation of aromatic α-hydroxy ketones or later discovered the catalytic effect of acetaldehyde on another synthesis.
What was new about List and MacMillan’s work, however, was asymmetric synthesis with organic catalysts. By “asymmetric,” it means reactions that selectively lead to only one of two possible enantiomers. These mirror-image isomer pairs exist, for example, when a molecule contains a stereocenter. Syntheses that selectively lead primarily to one enantiomer are often required for substances that are to be used in biological systems, such as drugs, pest control agents, or fragrances and flavorings.
The reason for this is that often only one of the two enantiomers has the desired effect, for example, a pharmaceutical effect or a certain smell and taste. According to a 2017 estimate, about every second substance used in medicine has a stereocenter. However, by no means do all manufacturers succeed in enantioselective synthesis.
Spatial control is what counts
The requirements for an asymmetric catalyst are high. It is not enough if it merely lowers the activation energy. In order to have an enantioselective effect on the course of the reaction, it must create a very specific spatial environment for the molecules involved in the reaction. This is the only way to ensure, for example, that the attachment of one reaction partner to the other can only take place from one side because the other is shielded.
In the case of enzymes, the complex structure of the protein molecule provides this “steric control.” In the case of catalysts based on transition metals, the effect is achieved by surrounding the metal atoms beforehand with suitable molecular frameworks, also known as ligands. In both cases, for spatial (steric) reasons, the decisive reaction leads preferentially to only one enantiomer, while the formation of the mirror image molecule is significantly more difficult.
It also works with small organic molecules
Not so long ago, it was assumed that only transition metal complexes and enzymes were suitable for asymmetric catalysts. It was not until the 2021 Nobel Prize winners in Chemistry discovered and proved that small organic molecules such as proline are also suitable for this purpose. Max Planck chemist Benjamin List initially researched protein catalysts based on antibodies. Crystal structure analyses revealed that both an amino and an acid group of the antibody were involved in the catalysis.
List wondered, might a simple organic molecule have the same effect if it has an amino group and an acid group? For example, an amino acid? In his first experiment, he tested whether the natural amino acid L-proline could enantioselectively catalyze the aldol reaction of acetone with aldehydes. It succeeded: the enamine state formed in the process by ketone and proline did indeed undergo spatially controlled reactions with aldehydes.
Only a little more than 20 years have passed since organocatalysis was first developed. During that time, the field of organocatalysis developed rapidly.
Cheaper, more environmentally friendly, and simpler
Many research groups around the world are now working on organocatalysis. Unlike most metal compounds, organocatalysts are generally simpler, can in principle be produced from sustainable raw materials, and are usually cheaper in price and non-toxic.
Transition metals, on the other hand, often have to be extracted under ecologically difficult conditions from ore deposits that are in any case limited and sometimes difficult to access. And in the best case, the reaction conditions are also moderate. The aldol reaction with proline, for example, takes just a few hours at room temperature. Reasons enough, then, to accelerate as many chemical reactions as possible with the aid of organic catalysts.
Why “mirror image” separation is important
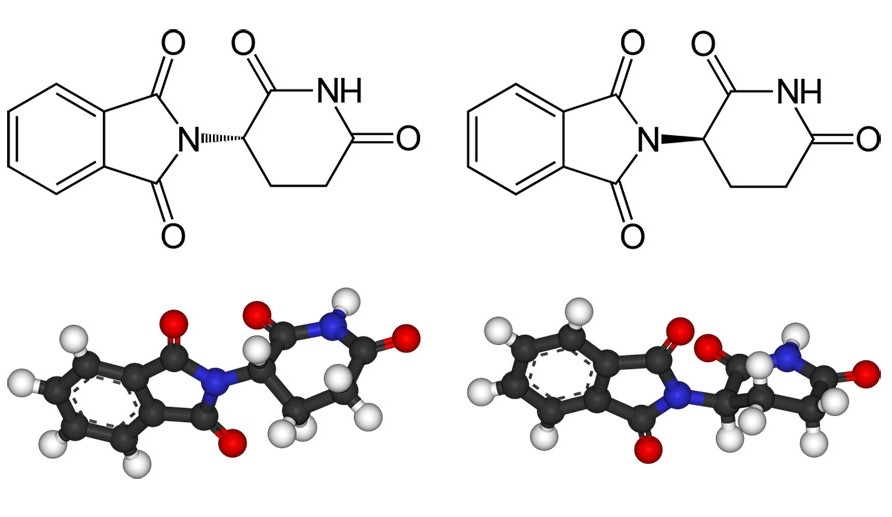
The fact that these are also suitable in principle for asymmetric catalysts has particularly spurred research. After all, these reactions often cause headaches for industry.
If the mirror-image products of a reaction cannot be controlled, this has two disadvantages. First, it reduces the yield of the desired enantiomer if some of the starting materials react to form the undesired isomer. Second, it takes effort, energy, and expense to separate the desired enantiomer from its mirror image. Manufacturers strive to make the synthesis as selective as possible.
However, there are still cases where enantioselective synthesis has not been successful at all, and manufacturers have to accept lower yields and enantiomer separation. For the sake of simplicity, many drugs contain the racemate, i.e., the mixture of both enantiomers. Of course, this is only possible if the undesired variant of the molecule is harmless.
Successful examples
Against this background, organic catalysts can certainly set new trends. One example is the synthesis of sitagliptin, an active ingredient in drugs for the treatment of type 2 diabetes. For the final synthesis step, enantioselective hydrogenation, a solution using an organic catalyst known as a dithiomalonate has also been available for some years, avoiding the technical challenges of using the metal catalysts commonly used in the past.
Another example is the reaction of hydrocyanic acid with 2-butanone, which leads to an important precursor of some active pharmaceutical ingredients. For a long time, there was no appreciable enantioselective route. In 2022, Benjamin List and his team published for the first time an organic catalysis with high enantioselectivity. The catalyst molecule is significantly larger and more complex than proline. According to List, this is now common and is partly because it gives us greater spatial control over reactions.
Today’s catalyst molecules are specifically designed to work much more effectively, so you need fewer of them. Whereas proline still had to be added to the reaction partners in concentrations of more than 20 percent for the aldol reaction, less than one percent, and in some cases significantly less, is sufficient for many modern organocatalysts.
Magic wand of chemistry
For Benjamin List, the fact that, in extreme cases, a single catalyst molecule is sufficient to manufacture products on a ton scale is precisely what fascinates him about his field of work. He also likes to talk about “magic molecules” because they can certainly be compared to “the magic wand” of a magician. The magician transforms objects with it, the chemists transform molecules.
Catalysis as a helper in climate protection and energy transition
Together with his team, Benjamin List, is working tirelessly on further catalysis innovations, certainly also with the aim of profoundly changing chemistry. Among other things, List hopes that many important chemicals can be synthesized more easily and in fewer reaction steps in the future, perhaps even directly from the components of crude oil, which for the time being remains an irreplaceable raw material for many chemical products.
The current challenges facing mankind, such as climate change or energy conversion, will only be solved with the help of catalysis.
To capture CO2
With regard to the most important greenhouse gas, carbon dioxide, he personally has a very special vision. In bold moments, he dreams of simply extracting this gas from the atmosphere and using UV light to break it down directly into carbon and oxygen. “You could bury the carbon,” List muses. But a prerequisite for the whole scenario would first be a suitable catalyst that cracks the strong double bonds between carbon and the two oxygen atoms without directly forming new bonds.
Nevertheless, it will not be possible to find an organic catalyst for every reaction. And where it does exist, it does not automatically have to be the better solution. There are cases where metal catalysts are almost impossible to replace. But scientists consider organocatalysis to be an important additional tool in the repertoire. Metals and enzymes nevertheless retain their purpose in catalysis.
As a rule, however, organic molecules often have clear advantages, such as greater sustainability, if they are even close to the same level of efficiency. Ultimately, it is also a matter of becoming “greener” for the entire chemical industry. That means working with processes that require less energy, are based on raw materials that are as sustainable as possible, and generate hardly any waste.